Essentials of Veterinary Ophthalmology. Kirk N. Gelatt
Чтение книги онлайн.
Читать онлайн книгу Essentials of Veterinary Ophthalmology - Kirk N. Gelatt страница 49
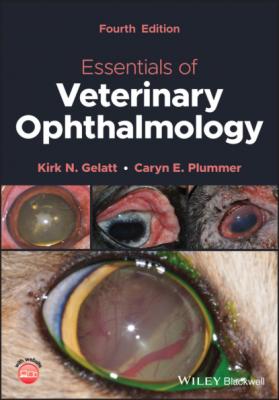
Cornea
The clear cornea serves as a window for the eye with two critical optical properties, transparency and refractive power, both of which are essential for vision. The cornea, with the sclera, protects the inner components of the eye from injury through its exquisite structure, biomechanics, and sensitivity.
Transparency
The cornea serves as the most powerful refractive structure of the eye. Corneal clarity or transparency is a result of the lattice‐like organization of the stromal collagen fibrils as well as the transparency of the cells within the cornea (Figure 2.2). The state of relative dehydration, hypocellularity, unmyelinated nerve fibers, a nonkeratinized epithelium, and absence of blood vessels and pigment also contribute to corneal transparency. The corneal stroma comprises the bulk of the cornea and is responsible for 90% of its thickness. It is predominantly composed of water that is stabilized by an organized network of collagens, glycosaminoglycans (GAGs), and glycoproteins. The GAGs are important for maintaining the regular spacing between fibrils. The uniform thickness, small collagen fibrils arrange into parallel lamellae running at oblique angles to each other, and are separated by less than a wavelength of light (Figure 2.3). This formation results in a highly ordered, lattice‐like arrangement whereby short‐range order results in corneal transparency via destructive interference.
Figure 2.2 In the normal cornea (a), a cross section of the corneal fibrils demonstrates a nearly perfect lattice arrangement, with equidistant collagen fibrils permitting light transmission and concomitant transparency. In contrast, swelling of the cornea with edema (b) disrupts this highly ordered arrangement, resulting in light diffraction and an opaque, bluish cornea.
Quiescent keratocytes lie between collagenous lamellae to form a closed, exquisitely structured syncytium. These three‐dimensional, stellate‐shaped cells comprise a cell body with multiple, extensive dendritic processes that interact with other keratocytes. Abundant corneal crystallins (~25–30% of the intracellular soluble protein), such as aldehyde dehydrogenase and transketolase, minimize refractive differences in the keratocyte cytoplasm, thus ensuring transparency of these cells.
Figure 2.3 Schematic of collagen fiber organization in the canine cornea. The epithelium produces an anterior basement membrane with a complex surface topography consisting of a meshwork of fibers and holes. The anterior 10% of the cornea comprises unidirectional, interwoven collagen lamellae, while the posterior 90% consists of unidirectional, nonwoven collagen lamellae with a random orientation. Descemet's membrane, the specialized basement membrane of the endothelium, can be divided into the anterior banded and posterior nonbanded layers. The anterior banded layer is dominated by collagen VIII, which appears as a hexagonal network en face and parallel bands in transverse section. The surface topography posterior nonbanded layer has a rich network of intertwined fibers, but with a smaller pore size in comparison to the anterior basement membrane.
Upon corneal wounding, transformation of keratocytes to activated fibroblasts and myofibroblasts results in a dramatic increase in cell volume and subsequent dilution of corneal crystallins, with a concomitant increase in light scatter. Corneal scarring is thought to be due to alterations in the light scattering properties of keratocytes, in addition to changes to the extracellular matrix (ECM).
The epithelium and endothelium are responsible for maintaining the cornea in a relatively dehydrated state. Specifically, loss of the corneal epithelium or endothelium results in a 200% or 500% increase in corneal thickness, respectively, due to stromal edema. Anatomical integrity of the epithelium and endothelium provides two‐way physical barriers against the influx of tears and AH, respectively. However, the multiple‐layered epithelium provides a relatively impermeable barrier versus the leaky, single‐layered endothelium. The endothelium primarily maintains stromal deturgescence via active transport that is energetically maintained by sodium–potassium‐activated adenosine triphosphatases (Na+–K+‐ATPases).
Metabolism
Steady‐state hydration in the cornea occurs when the endothelial leak and pump rates are equivalent; this process is termed the “pump–leak” mechanism. The leaky barrier function of the endothelium may at first seem counterintuitive, but most nutrients for the cornea, except oxygen, come from the AH. Thus, leakiness of the endothelium is essential to providing bulk fluid flow through a tissue that lacks blood and lymphatic vessels. Glucose transporters are found on both the apical and basolateral endothelial cell membranes that face the AH and stroma, respectively, to ensure transcellular glucose flux. The corneal epithelium converts glucose to glucose 6‐phosphate, where it is subsequently metabolized to pyruvate via glycolysis. Most of this pyruvate is then metabolized into lactate, but some is diverted into the tricarboxylic acid cycle to produce ATP. Glucose is also stored in the epithelium as glycogen, which can be used for energy under stressful conditions such as corneal injury. The corneal epithelium and keratocytes in the anterior stroma obtain oxygen for aerobic glycolysis from the PTF, while the endothelium and keratocytes in the posterior stroma receive their oxygen from the AH.
Biomechanics
The cornea is a thick‐walled, pressurized, partially intertwined, unidirectionally fibril‐reinforced laminate biocomposite, which imparts stiffness, strength, and extensibility to withstand both inner and outer forces that may alter its shape or integrity. A soft, fibrous connective tissue, like the cornea, usually is much stronger in the parallel versus perpendicular direction to the collagen fibrils. Consequently, the collagen fibrils are arranged into complex hierarchic structures, which give the cornea its anisotropic mechanical properties. The collagen lamellar architecture of the cornea varies dramatically between vertebrate species, with nonmammalian vertebrates exhibiting an orthogonal‐rotation arrangement with a marked increase in lamellar branching in species such that birds >> reptiles > amphibians > fish; in contrast, the mammalian species exhibit a random pattern.
Tissues are biomechanically characterized by measuring the elastic modulus, a property that defines a material's ability to resist deformation under an applied stress, which approximates its stiffness. The elastic moduli of the corneal layers as measured by atomic force microscopy have been reported in the human and the rabbit (Table 2.3); all layers of the human cornea were stiffer than those of the rabbit. This variability in corneal collagen fiber organization and matrix properties between species likely contributes to their diverse mechanical properties, and may influence indentation tonometry (estimation of intraocular pressure [IOP]).
Sensitivity and Innervation
The cornea is an exquisitely sensitive tissue, and this sensitivity provides a critical protective function. Upon stimulation of the cornea, involuntary blinking occurs via intermediate relays from the ophthalmic branch of the trigeminal nerve to orbicularis oculi innervation from the facial nerve