Musculoskeletal Disorders. Sean Gallagher
Чтение книги онлайн.
Читать онлайн книгу Musculoskeletal Disorders - Sean Gallagher страница 36
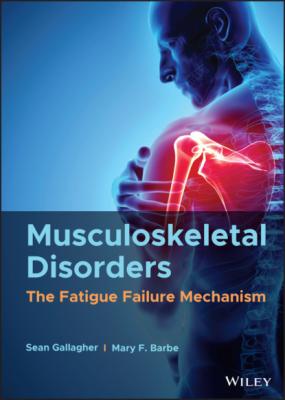
Myosin filament sliding
The contraction of muscle tissues provides motion, maintenance of posture, and heat production. The mechanisms of contraction of sarcomeres are key to muscle function. The thin and thick filaments only partially overlap in resting sarcomeres (Figure 3.7). However, during contraction, the amount of overlap between these filaments increases due to sliding of the filaments past one another (Figure 3.10), which causes shortening of the sarcomere. This contraction is induced by an action potential produced at the myoneural synapse (the juncture of a somatic motor axon and a muscle fiber). At the sarcomere level, during contraction, a small subset of myosin heads align with available actin‐binding sites. As the bound myosin heads move the actin, they provide for alignment of new actin–myosin cross bridges. The old bridges detach only after the myosin binds a new ATP molecule. This action resets the myosin head and prepares it for another contraction cycle. If no ATP is available, the actin–myosin complex become stable, which accounts for the extreme muscle rigidity associated with death (rigor mortis). A single muscle contraction is the result of hundreds of bridge‐forming and bridge‐breaking cycles. The contraction activity that leads to a complete overlap between thin and thick filaments continues until Ca2+ ions are removed, and the troponin–tropomyosin complex again covers the myosin‐binding site.
Neuromuscular junction and muscle contraction
Skeletal muscle is considered as a voluntary muscle because it can be made to contract by conscious controls. In order for a skeletal muscle fiber to contact, it must first be stimulated by an impulse (an action potential) from a motor nerve cell. The neurons that stimulate muscles to contract are somatic (of the body) motor neurons and have cell bodies that originate in the spinal cord or brainstem and have nerve processes (axons) that extend into the periphery to muscles (see Chapter 4). In skeletal muscle, myelinated somatic motor nerves branch into the perimysium and divide into several terminal smaller branches that innervate the muscle fibers. At the site of innervation, the axon loses its myelin sheath and forms a dilated terminal situated within a trough on the muscle cell surface, the neuromuscular junction (also known as a motor end plate) (Figure 3.11). Within this axon terminal are numerous synaptic vesicles containing the neurotransmitter acetylcholine. When a somatic neuron fires and the action potential reaches the motor end plate, acetylcholine is released into a synaptic cleft (a space between the axonal terminal and the muscle). The synaptic cleft is a highly folded region of the sarcolemma that allows for more surface area. There are numerous mitochondria, ribosomes, and glycogen granules at this site. When acetylcholine binds to its receptor, Na+ channels open and membrane depolarization results. Excess acetylcholine in the synaptic cleft is hydrolyzed by the enzyme cholinesterase to avoid prolonged contact of the neurotransmitter with its receptors. The depolarization is then propagated deep into the myofiber by the previously discussed transverse tubule system. At each triad (a T‐tubule and two cisterna), the depolarization signal is passed to the sarcolemma reticulum. This results in a release of Ca2+ and initiation of the muscle cells’s contraction cycle. When the depolarization ceases, the Ca2+ is actively transported back into the cisterna for storage, and the muscle cell relaxes.
Figure 3.10 The sliding‐filament mechanism.
Tortora, G. J., & Derrickson, B. H. (Eds.), (2010). Muscle. In Introduction to the human body, 11th ed., Wiley.
Extracellular matrix/fascia
As previously mentioned and depicted (Figures 3.3 and 3.6), individual muscle fibers are bound together by a complex system of collagenous supporting tissue that link individual myofibers into a fascicle in order to form a single functional mass (Gillies & Lieber, 2011). The size of the fasciculi reflects the function of a muscle. Muscles responsible for fine controlled movements, like those of the hand, have small fasciculi and a relatively greater proportion of perimysial supporting tissue. Larger muscles responsible for gross movements have large fascicles and relatively little perimysial tissue. Muscle fibers are also anchored by these fascial connective tissues. The external lamina transmits the contractile forces developed by the internal contractile proteins. The connective tissue framework becomes continuous with those of tendons, as well as bone or skin when muscles are directly attached to these latter structures. The extracellular matrix also serves as a biological reservoir of muscle stem cells. In both injured and diseased states, the extracellular matrix adapts dramatically, a property that has clinical manifestations for muscle function.
Figure 3.11 The neuromuscular junction. Adult skeletal muscle is highly organized with one neuronal branch innervating each myofiber at the neuromuscular junction, which exhibits a mature, pretzel morphology with direct overlap between the presynaptic nerve terminal bouton and the postsynaptic acetylcholine receptor clusters.
Gilbert‐Honick, J. & Grayson, W. (2020). Vascularized and innervated skeletal muscle tissue engineering. Advanced Healthcare Materials 9(1): e1900626. Wiley.
Muscle as an endocrine system
Skeletal muscle is also an endocrine system and serves as a major site of insulin‐stimulated glucose disposal and homeostasis (Mukund & Subramaniam, 2019). It secretes a variety of cytokines and peptides, termed “myokines,” including interleukin (IL)‐8, IL‐18, IL‐15, insulin like growth factor 1 (IGF1), and fibroblast growth factor 21 (FGF21). These factors are regulated by muscle contractile activity and exercise. These myokines exert autocrine (self), paracrine (related only to the local vicinity of the organ producing the factor), and endocrine (systemic signaling) effects in a context‐dependent manner that enables muscles to maintain metabolic homeostasis during health and exercise, and responses during chronic metabolic conditions and disorders. This will be discussed further in Chapters 11 and 15.
Tendon
Tendons are a type of dense regular connective tissue since they are arranged in a highly organized pattern with a clear predominance of collagen fibers over ground substance and cell numbers. They can also be thought of as a fibrous cord or band of variable length that anchor muscles to bone. Many muscles have two tendons at both their proximal and distal ends (such as the biceps muscle of the upper extremity), although some muscles attach directly to bone without a tendon (such as the proximal attachments of deep flexor and extensor muscles on the radius and ulna). Tendons may unit with muscles at the end of a muscle, or it may run along the side of the muscle or within its center for long or short distances, receiving muscle fibers along its lateral border. The point of union with a muscle is called a myotendinous junction (Kannus, 2000). Although tendons are characterized by their great tensile strength (Benjamin & Ralphs,