Biogeography in the Sub-Arctic. Группа авторов
Чтение книги онлайн.
Читать онлайн книгу Biogeography in the Sub-Arctic - Группа авторов страница 13
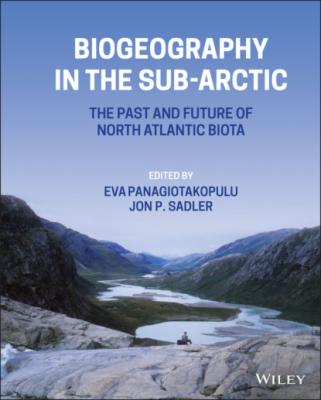
The spreading process confers bilateral symmetry to the mid‐ocean ridges as juvenile material is welded on either side to the pre‐existing lithosphere. The mantle rocks that are not consumed in the melting remain behind, contributing to the lower part of the oceanic lithosphere. Sea‐floor spreading occurs at rates that can exceed 100 mm/year, whilst that in the North Atlantic is roughly 25 mm/year (Bown and White 1994).
When extensional stresses acting on a ridge sector become too great, rupturing (rifting) occurs along its crest and the new‐formed magma rises up a vertical fissure to form an intrusive ‘dyke’. The dykes cool to form tabular, near‐vertical intrusions, typically not more than a few metres wide. Their repeated generation produces a ‘sheeted complex’ in which the youngest are those newly formed along the spreading axis and the oldest are those furthest away. Dykes can compose virtually the entirety of the sheeted complexes lying beneath the lavas extruded on the ocean floor.
Figure 1 The bathymetry of the North Atlantic, based on satellite sea‐surface altimetry, model DNSC08. The symmetrical disposition of the mid‐Atlantic ridge relative to the bounding continents is well exhibited.
Source: Based on Anderson, O.B. & Knudsen, P. 2009.
Because of this mechanism the spreading (and thus growth of the oceanic plate) does not take place continuously but in a jerky, spasmodic manner. For example, generation of a new fissure 1 m wide every 50 years would correspond to an averaged spreading rate of 20 mm/year. Hence, whilst the spreading ocean floors can be crudely considered as analogous to moving walkways, it is intermittent with intervals of tens to hundreds of years intervening between the intrusion of one dyke and the next. The relatively hot rocks composing the axial region have a lower density so that they rise to form the mid‐ocean ridges. With age and cooling they subside to the depths of the abyssal plains.
The mid‐ocean ridges are offset by faults of a type known as transform faults. The motions along these fractures (which can have offsets ranging up to hundreds of kilometres) are dominantly horizontal. In the North Atlantic there are several of these major fractures that subdivide the mid‐ocean ridge between Greenland and Norway. The most prominent are the Senja fracture zone to the south of Spitzbergen and the Jan Mayen fracture zone at ~52°N.
Magnetic Anomalies
As a result of processes in the Earth's core, the magnetic field spontaneously reverses at irregular intervals averaging at 500,000 years. The direction of magnetization is recorded within the oxide minerals that crystallize from the magmas, much as in a compass needle. As they cool, these minerals retain a memory of the field at the time of their formation. As sea‐floor spreading proceeds, alternating strips of ‘normally magnetized’ (i.e. with the magnetic field as it is currently) and those with ‘reversed magnetization’ are left on the ocean floor (Figure 2, see Plate section). It was the discovery of these ocean floor magnetic anomalies by Vine and Matthews (1963) that provided confirmation of Hess's (1962) ocean‐floor spreading hypothesis. Vine and Matthews demonstrated that continuous growth of the ocean floor, in conjunction with the periodic reversals, produces distinct magnetic stripes, developed as mirror‐images on either side of the spreading ridge.
These magnetic signatures record the past positions of the ridge axis and, since specific ages can now be assigned to the reversals, the age of the ocean floor from new‐formed crust to oldest (first‐formed) crust can be determined.
Mantle Plumes
Masses of mantle rock rise convectively when their composition and/or temperature confers buoyancy. Temperature, however, is regarded as the dominant factor and great volumes of abnormally hot rock are thought to detach periodically from deeper parts of the mantle to rise as so‐called mantle plumes. Although the concept of mantle plumes dates back to the early 1970s, it remains controversial with opponents of the idea referring simply to foci of high temperature phenomena as ‘hot‐spots’ and denying that such plumes arise from the deep mantle (e.g. Anderson 2005).
The idea that mantle plumes have a mushroom‐like shape, with a massive plume head and an extremely hot tail, is based on fluid‐dynamical studies. The most voluminous magmatic events during the Earth's history have been related in space and time to an impact at the base of the lithosphere by such a plume head. Such a ‘hot‐spot’ model was outlined for the North Atlantic by White (1988), postulating a central plume causing raised asthenospheric temperatures across a wide region. A review of conflicting hypotheses with respect to the North Atlantic by Meyer et al. (2007) concluded that it would be difficult to present a model explaining both the records of igneous activity and associated uplift (discussed below) without appealing to an up‐rise of hot mantle beneath the lithosphere. Because the various arguments cannot be rehearsed in this chapter the author adopts a partisan attitude, regarding the plume model as the more robust and better supported by evidence.
The Iceland Plume
The sudden and massive onset of magmatic activity in what may be broadly termed the north Atlantic region during the early Cenozoic (i.e. in the Palaeocene and Eocene epochs, between 66 and 34 Ma) has been attributed to a dramatic increase in the temperature of the underlying mantle starting at around 62 Ma. The most plausible explanation for this is that it was due to the arrival at shallow levels of an abnormally hot mantle body, namely a mantle plume. However, some sea‐mounts in the Rockall Trough, dated at 70+/−1 Ma, may indicate some precursor plume activity commencing in the Cretaceous (O'Connor et al. 2000).
Figure 2 The pattern of magnetic stripes in the North Atlantic, 2005. Geological Map of the World. Scale: 1 : 50 000 000.
Source: Published by CGMW & UNESCO.
The opening of the North Atlantic (and the subsidiary opening of Baffin Bay west of Greenland) is believed to have involved complex interaction between this plume (the proto‐Iceland plume evolving to the Iceland plume) and ‘normal’ sea‐floor spreading processes (White 1997; White and McKenzie 1989; Fitton and Larsen 2001; Smallwood and White 2002). The abundant generation of magma gave rise to a great igneous province prior to and accompanying the sundering of Laurussia and the creation of the embryonic North Atlantic Ocean. This province embraced a large region including eastern Greenland, a large part of the Norwegian shelf (the Vøring Plateau), the Faeroes and the Hebridean and Northern Irish parts of the British Isles. The cause of this magmatic activity is held to be partial melting generated by the proto‐Iceland plume as is the, approximately contemporaneous, activity that occurred across part of western Greenland and eastern Baffin Island. The magmatism commenced abruptly and was also relatively short‐lived, being mostly confined within 2–3 million years (White 1988). Not only were prodigious quantities of basaltic magma produced but also unusually magnesian (‘picritic’) magmas signifying exceptionally high temperatures were erupted, mainly in the opening stages of the activity. The time interval between the start of continental lithosphere stretching and creation of embryonic ocean was short, perhaps only 4–6 Ma (Smallwood and White 2002). This suggests that injection of great volumes of magma into