Molecular Mechanisms of Photosynthesis. Robert E. Blankenship
Чтение книги онлайн.
Читать онлайн книгу Molecular Mechanisms of Photosynthesis - Robert E. Blankenship страница 14
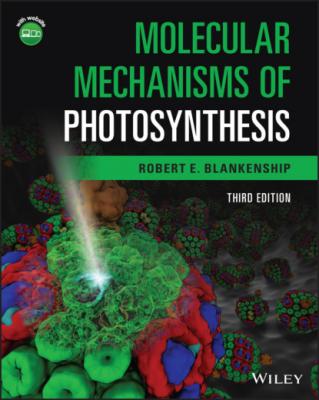
Protons are also transported across the membrane and into the thylakoid lumen during the process of the noncyclic electron transfer, creating a protonmotive force. The energy in this protonmotive force is used to make ATP (see Chapter 8).
Reaction centers and electron transfer processes in anoxygenic bacteria are discussed in more detail in Chapter 6, while these processes in oxygenic photosynthetic organisms are discussed in more detail in Chapter 7.
1.4.4 Synthesis and export of stable products
The final phase of photosynthetic energy storage involves the production of stable high‐energy molecules and their utilization to power a variety of cellular processes. This phase uses the intermediate reduced compound, NADPH, generated by Photosystem 1, along with the phosphate bond energy of ATP to reduce carbon dioxide to sugars. In eukaryotic photosynthetic organisms, phosphorylated sugars are then exported from the chloroplast. The carbon assimilation and reduction reactions are enzyme‐catalyzed processes that take place in the chloroplast stroma. These reactions are discussed in more detail in Chapter 9.
References
1 Béjà, O., Aravind, L., Koonin, E. V., Suzuki, M. T., Hadd, A., Nguyen, L. P., Jovanovich, S., Gates, C. M., Feldman, R. A., Spudich, J. L., Spudich, E. N., and DeLong, E. F. (2000) Bacterial rhodopsin: Evidence for a new type of phototrophy in the sea. Science 289: 1902–1906.
2 Ernst, O. P., Lodowski, D. T., Elstner, M., Hegemann, P., Brown, L. S., and Kandori, H. (2014) Microbial and animal rhodopsins: Structures, functions, and molecular mechanisms. Chemical Reviews 114: 126–163
3 Falkowski, P. and Raven, J. (2007) Aquatic Photosynthesis, 2nd Edn. Princeton, NJ: Princeton Univerity Press.
4 Gómez‐Consarnau, L., Raven, J. A., Levine, N. M., Cutter, L. S., Wang, D., Seegers, B, Arístegui, J., Fuhrman, J. A., Gasol, J. M., and Sañudo‐Wilhelmy, S. A. (2019) Microbial rhodopsins are major contributors to the solar energy captured in the sea. Science Advances 5: eaaw8855.
5 Hohmann‐Marriott, M. F. and Blankenship, R. E. (2011) Evolution of photosynthesis. Annual Review of Plant Biology 62: 515–548.
6 Inoue, K., Tsunoda, S. P., Singh, M., Tomida, S., Hososhima, S., Konno, M., Nakamura, R., Watanabe, H., Bulzu, P.‐A., Banciu, H., Andrei, A.‐S., Uchihashi, T., Ghai, R., Béjà, O., and Kandori, H. (2020) Schizorhodopsins: A family of rhodopsins from Asgard archaea that function as light‐driven inward H+ pumps. Science Advances 6: eaaz2441.
7 Kiang, N., Siefert, J., Govindjee, and Blankenship, R. E. (2007a) Spectral signatures of photosynthesis. I. Review of earth organisms. Astrobiology 7: 222–251.
8 Kiang, N., Segura, A., Tinetti, G., Govindjee, Blankenship, R. E., Cohen, M., Siefert, J., Crisp, D., and Meadows, V. S. (2007b) Spectral signatures of photosynthesis. II. Coevolution with other stars and the atmosphere on extrasolar worlds. Astrobiology 7: 252–274.
9 Kirk, J. T. O. (2011) Light and Photosynthesis in Aquatic Ecosystems, 3rd Edn. Cambridge: Cambridge University Press.
10 Pushkarev, A., Inoue, K., Larom, S., Flores‐Uribe, J., Singh, M., Konno, M., Tomida, S., Ito, S., Nakamura, R., Tsunoda, S. P., Philosof, A., Sharon, I., Yutin, N., Koonin, E. V., Kandori, H., and Béjà, O. (2018) A distinct abundant group of microbial rhodopsins discovered using functional metagenomics. Nature 558: 595–599
11 Schweiterman, E. W., Kiang, N. Y., Parenteau, M. N., Harman, C. E., DasSarma, S., Fisher, T. M., Arney, G. N., Hartnett, H. E., Reinhard, C. T., Olson, S. L., Meadows, V. S., Cockell, C. S., Walker, S. I., Grenfell, J. L., Hegde, S., Rugheimer, S., Hu, R., and Lyons, T. W. (2018) Exoplanet biosignatures: A review of remotely detectable signs of life. Astrobiology 18: 663–708.
12 Thekaekara, M. P. (1973) Extratesrrrestrial solar spectral irradiance. In: A. J. Drummond and M. P. Thekaekara, (eds.) The Extraterrestrial Solar Spectrum. Mount Prospect, IL: Institution of Environmental Sciences, pp. 71–133.
Chapter 2 Photosynthetic organisms and organelles
2.1 Introduction
Green is all around us. The distinctive color of chlorophyll announces the presence of photosynthetic organisms, including trees, shrubs, grasses, mosses, cacti, ferns, and many other types of vegetation. But this is just the tip of the iceberg of photosynthetic life. In addition to these most visible organisms, there is a remarkable variety of microscopic life, including many types of algae and bacteria that carry out photosynthesis. This chapter will introduce the different types of photosynthetic and phototrophic organisms and will give some information about their cellular organization and structure.
All living things on Earth are related to each other. In some cases, the relationships are obviously close, such as between a dog and a coyote, or an orange tree and a lemon tree, while in other cases the relationships are apparent only upon close examination, such as between a bacterium and a human or an amoeba and a fish. To establish these less obvious relationships, it is necessary to look at a deeper level of analysis, down to the cellular and even the molecular levels (Alberts et al., 2014; Nelson and Cox, 2017). At these levels of organization, the unity of life is readily apparent. All organisms are organized in the same fundamental way, with DNA serving as the master copy of the information needed to construct the organism, RNA as the intermediate working copy, and proteins as the workhorses of the cell, carrying out almost all the chemical reactions that make up metabolism. This basic pattern of information flow and metabolic responsibilities is known as the central dogma of molecular biology. Although some exceptions are known, such as viruses that use RNA for information storage or RNA molecules that act as enzymes, the basic pattern applies to all life. The chemical structures of the building blocks of DNA, RNA, and proteins are exactly the same in bacteria and humans. The process of copying DNA into RNA is called transcription, and the translation of the nucleic acid code into proteins is called translation. This latter process takes place on large protein‐RNA complexes called ribosomes.
Cells are surrounded by membranes, which function as permeability barriers and also carry out many important functions. Membranes are composed of lipids, which are amphipathic molecules with a polar head group and nonpolar tail. The lipids are arranged in a bilayer structure, with the polar head groups toward the outside and inside of the cell, and the nonpolar tails pointed into the center of the bilayer. There are many types of lipids, which form several classes. Two of the most important are phospholipids and glycolipids. Phospholipids include a phosphate group that is esterified to a glycerol backbone. The most common type of lipids in chloroplasts are glycolipids, in which sugars are found in place of the phosphate groups. The nonpolar tails are long‐chain fatty acids that are esterified to the glycerol groups. They almost always contain one or more double bonds, which increase the fluidity of the membranes of which they are the principal components. The cell membrane is often called the cytoplasmic membrane, while the space enclosed is