Smart Grid Telecommunications. Ramon Ferrús
Чтение книги онлайн.
Читать онлайн книгу Smart Grid Telecommunications - Ramon Ferrús страница 25
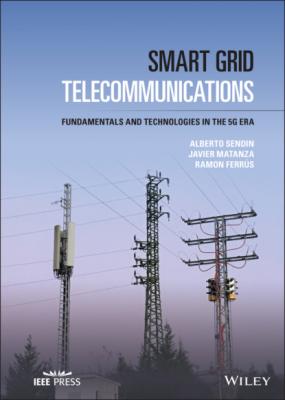
A group of very relevant requirements refers to EMC. EMC collates different groups of aspects: radiated and conducted emissions, immunity to radiated and conducted disturbances, insulation, electrostatic discharge immunity, electrical fast transient/burst immunity, surge immunity, voltage‐dips/interruption immunity, etc. For conducted or radiated emission limits CISPR 32 is usually considered. Immunity requirements may be taken from tests proposed in IEC 61000‐4 series.
The rest of non‐functional requirements fall in the environmental category. This group refers to climatic and mechanical (vibration, shock, seismic) conditions, to be taken into account in the product lifecycle (storage, transportation, and in normal‐use regime) according to their different environmental conditions (weather‐protected, temperature control; stationary use, mobile use, portable use, etc.). There is a very complete reference in Europe in ETSI 300 019 series. The tests for the different classes are based on IEC 60068‐2 series “Environmental Testing – Part 2: Tests.” Alternatively, a much simpler reference is IEEE 1613.1. IK codes (IEC 62262) and IP codes (IEC 60529) for protection against ingress of solid foreign objects and against ingress of water with harmful effects need also to be taken into consideration, although these are not very different from other fields. Recommended limits for all these aspects can be found in [34].
There is a very important aspect that influences the relationship between telecommunications and the substation environment, and it has to do with earthing/grounding aspects [61]. Substations protect all elements inside them from unexpected HV events such as power faults and lightning strikes (anything that may cause a discharge of large amounts of electrical energy into its surroundings) by providing an equal‐potential zone with its ground grid. The substation ground grid connects all metal parts together to create an equipotential zone, so that everything within the compound is at the same potential. While this creates a safety mechanism for the substation, it will affect any externally connected metallic cable, if it is not properly engineered. This situation has been widely studied for PSs in IEEE 367‐2012, IEEE 487‐2015, and IEEE 1590‐2009, and although HV insulators have been developed to allow for the safe connection of, e.g., copper pairs reaching the substation from TSPs premises, the installation of optical fiber in dielectric cables, or radio‐based solutions, is preferred to connect substations. The situation is similar in SSs; although distances within SSs are much smaller, the MV ground reference is different to the LV ground reference of the neutral wire, and this imposes extra insulation requirements in devices connected to the MV and LV ground references, simultaneously.
1.6.7 Distributed Intelligence
There has been a recurrent discussion in utility industry around the IT and Telecommunication aspects of ICTs, when it comes to the availability of both in grid asset sites.
Computing power and telecommunications have been at large scarce resources. This fact, connected to the evolution of some traditional control system applications (i.e., SCADA), has configured different historical trends when deciding if system intelligence had to be distributed or centralized.
If we take the IT component, processing power has been expensive and bulky in the old times. At the same time, SCADAs started at power plants and PSs to automate operations locally (see Chapter 5) and that long‐distance Telecommunications were not readily accessible in many remote areas. These circumstances created an electric power system control structure that installed part of its computing power in distributed premises (substations).
However, the expansion of telecommunication capabilities, combined with the size reduction of computing power and the enhanced automation capabilities of evolving SCADAs, pushed a wave of SCADA system concentration that leveraged the cost savings produced by synergies in the SCADA operations. Thus, distributed intelligence started to diminish.
SCADA evolution was parallel to remote meter reading systems. The situation of these systems changed the perspective of the telecommunications access to substations. From the need to access a few PSs of early SCADAs, to the need to access many SSs where high‐throughput connectivity was not affordable, changed again the approach. At this point, the idea of “data concentrators” (see Chapter 5) appeared, as a way to downsize central MDM systems with a certain distributed intelligence.
Those two parallel stories of Smart Grid systems embryos, different in the use of ICT components, have reached our days, and numerous initiatives in Smart Grids show the influence of both.
In the telecommunications domain, the availability of high‐throughput connectivity is common today, and telecommunication bottlenecks are not to be expected in urban, suburban, or populated rural areas. Thus, the distributed intelligence concept is no longer generally a must. On the contrary, the general availability of communications to access all SSs leaves the decision on where to place computing power to utilities.
However, telecommunications themselves, and for their purpose of adapting to the various market needs, are witnessing two trends or types of systems in their evolution:
Internet of Things (IoT)‐type of connectivity, as a connectivity intended to provide some sort of low‐end telecommunication capabilities to any location.
Telecommunications network intelligence closer to the network end‐points, helping to provide low latency services that might be demanded by some users.
Thus, another level of complexity is added to the Smart Grid distributed intelligence discussion that should consider these new trends of telecommunications market.
1.6.8 Resilient Telecommunication Networks and Services
Two different concepts mix when referring to resiliency in telecommunications: reliability and resiliency. Before we refer to them, we need first to focus our attention on the availability concept, prevalent in the measurement of the service conditions.
Availability is defined in ITU‐T E.800 as the ability “of an item to be in the state to perform a required function at a given instant of time or at any instant of time within a given time interval, assuming that the external resources, if required, are provided.” When the network is available, its performance can be observed and measured. Many ITU‐T and ITU‐R recommendations define availability objectives. Few references, however, exist for Smart Grid‐related telecommunication services, although it can be broadly assumed that the strictest Smart Grid services need 99.999% availability (this means 315.36 seconds of unavailability during the year).
To have networks and services in the “available” state, reliability concept appears. It is defined in ITU‐T E.800 as “the probability that an item can perform a required function under stated conditions for a given time interval.” Reliability is different to resiliency. Resiliency can be defined as “the ability of an entity, system, network or service to resist the effects of a disruptive event, or to recover from the effects of a disruptive event to a normal or near‐normal state” [62]. Thus, while reliability focuses on preventing that the system fails, resilience focuses on recovering when it fails.
Resilience in telecommunications implies carrier‐grade (carrier‐class) network elements (equipment with redundancy in its vital parts – such as power supply), reliable design of network links (with the proper availability assurance), protection of paths through