Nanotechnology in Plant Growth Promotion and Protection. Группа авторов
Чтение книги онлайн.
Читать онлайн книгу Nanotechnology in Plant Growth Promotion and Protection - Группа авторов страница 27
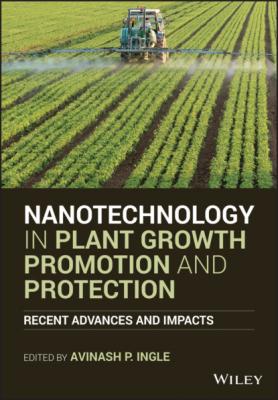
3 The Emerging Applications of Zinc‐Based Nanoparticles in Plant Growth Promotion
Anil Timilsina and Hao Chen
Department of Agriculture, University of Arkansas, Pine Bluff, AR, USA
3.1 Introduction
Zn nanoparticles (Zn NPs) are among the top three highest produced and utilized engineered nanoparticles (Zhang et al. 2015b). Variations in synthesis methods, such as preparation method, choice of precursors, treatment condition, and treatment duration, affect the properties of synthesized nanoparticles (Kołodziejczak‐Radzimska and Jesionowski 2014). To obtain stability, after synthesis Zn NPs are often undergo a coating process to get the final nanomaterial product. Depending on the shape, size, and aggregation type, the application and effect of nanoparticles vary.
In agriculture, Zn‐related nanomaterials are mostly used as nano‐fertilizer and nano‐pesticide. Zinc cations activate enzymes, such as RNA polymerase, superoxide dismutase (SOD), alcohol dehydrogenase, and carbonic anhydrase; they participate in photosynthesis and metabolism of carbohydrates, lipids, and nucleic acids (Sturikova et al. 2018). Zinc cations facilitate the transcription factors in plants that control the proliferation and differentiation of cells (Palmer and Guerinot 2009). Besides, zinc also controls the development of chloroplasts and some of their functions (Hänsch and Mendel 2009). Zinc deficiency in the plant often causes abnormal plant development and enzyme activity disruption, such as photosynthesis inhibition. In case of an extensive acute deficiency, plants often show dwarf growth and chlorosis (Sharma et al. 2013).
Zn NPs are beneficial because compared to bulk Zn (ZnSO4) they release plant‐available Zn2+ at higher concentration with longer time; therefore, Zn delivery efficiency is higher when applied to Zn in nanoparticle forms (Sturikova et al. 2018). The efficiency of NPs fertilizer or pesticides depends on the size, concentration, surface coatings of the NPs, application method, and the plant species. However, an excess amount of Zn can also be toxic to plants because Zn may compete with other biologically active ions (e.g. Fe2+ and Fe3+) for binding sites in the plant cell (Krämer 2010). Typical symptoms of zinc toxicity include chlorosis of young leaves, the reduction of plant biomass, and inhibition of root growth (Broadley et al. 2007). Therefore, the sustainable use of Zn NPs to promote plant growth is challenging. Considering these facts, the present chapter is aimed to focus on recent advances in the use of Zn NPs in plant growth promotion. Moreover, we believed that systematic studies on interactions between Zn‐based NPs and plants are needed to provide scientific application guidelines in the agricultural system.
3.2 Applications and Effects of Zn Based NPs on Plant Growth Promotion
3.2.1 Zn NPs in Seed Treatments and Its Effects
Recently, Zn NPs have attracted a great deal of attention from the scientific community around the globe for their use in seed treatment. Various seed treatment methods such as wet treatments, including seed coating and seed dipping are commonly used to improve seed health (Taylor et al. 2001). In the seed film coating process, NPs are often mixed with other substances such as clay then applied as a thin layer over the seed surface (Butler 1993; Taylor 1999). There are different methods are proposed to treat seed with Zn NPs (Figure 3.1). Seeds can be first soaked into Zn NPs suspension for a certain time and dried to an original moisture content of the seed. Besides, seeds can also be partially or completely dipped in a small amount of Zn NPs suspension for a certain time then followed by germination without drying. Lastly, seeds can be soaked in a large volume of Zn NPs suspension for the limited time period and allowed to germinate without drying (Panda 2017).
In general, seed priming requires a high concentration of ZnO (more than 500 mg/L). For example, maize seed treatment with ZnO NPs (25 nm) at 1500 mg/L was found optimum for seed germination (increased by 40%) and seedling vigor (root and shoot length increased by >50%) (Subbaiah et al. 2016). Elhaj Baddar and Unrine (2018) also reported positive effects of ZnO, ZnO core Zn3(PO4)2 shell or dextran‐coated ZnO NPs without adverse effects on wheat seedling growth with concentration about 500 mg/L. A study on peanuts also shows positive effects of nanoscale ZnO (25 nm) during germination, where 1000 mg/L treated seed produced the highest germination percentage and seed vigor index (Prasad et al. 2012). However, for certain crops, ZnO NPs can be effective at a concentration lower than 100 mg/L. Munir et al. (2018) found a linear increment in wheat seed germination and seedling growth after seed priming with ZnO NPs from 25 to 100 mg/L concentration. Similarly, Panda (2017) showed a linear increment of positive effects of Zn NPs from 5 to 50 mg/L (30–60 nm) on rice seed germination and enzyme activity through seed soaking for one hour with a significant increment in SOD and antioxidant enzymes (reductases).
3.2.2 Effects of Zn NPs on Seed Germination
Zn plays a critical role in the production and activation of germination enzymes (digestive and antioxidant enzymes) and hormones (auxin and gibberellin) that improve seed germination and seedling growth (Broadley et al. 2007). Seedling in Zn NPs suspension requires low Zn NPs concentration (<100 mg/L) for better seedling growth. Awasthi et al. (2017) found optimum effects on germination and seedling growth in wheat is germination at 50 mg/L ZnO NPs (9 nm) suspension. Zn NPs were found to be more dose effective in the case of smaller seeds like cabbage, onion, and lupine (Table 3.1). Pokhrel and Dubey (2013) examined the difference between the effects of ZnO NPs on cabbage and maize seed germination. The results obtained indicated that cabbage seeds with smaller size and more surface area to volume ratio are more vulnerable to the negative effect of ZnO NPs. They also reported that concentrations higher than100 mg/L showed a significant negative effect in cabbage seed germination. Whereas, in the case of maize, ZnO NPs showed positive effects in seed germination up to 500 mg/L. Raskar and Laware (2014) reported that ZnO NPs enhanced onion seed germination only at a concentration lower than 20 μg/mL. Similarly, Latef et al. (2017) found that ZnO NPs at 60 mg/L showed positive effects on lupine seed germination and seedling vigor. However, high concentration Zn NPs treatment to small size seeds for a long time period can be toxic. Black mustard grown at 500 mg/L ZnO NPs treated plain agar media for 12 days showed negative effects on germination and seedling growth (Zafar et al. 2016). Seed growth media also impact the dose efficiency and toxicity of Zn NPs. According to Thunugunta et al. (2018), 100 mg/L ZnO NPs (35 nm) showed positive effects on eggplant seed germination when amended to soil; however, concentration as little as 5 mg/L showed adverse effects when applied through Murashige and Skoog (MS) media. Table 3.1 shows the effects of Zn NPs on germination and seedling growth after application through different modes.
Figure 3.1 Outline of different methods used for the treatment of seeds with Zn NPs and their effects.
Table 3.1 Effects of Zn NPs on germination and seedling growth after application through different modes.
Size and coatings (nm) | Crop | Concentrations | Mode of application | Effects | References |
---|---|---|---|---|---|
Root growth | Shoot growth |
|