Plastics and the Ocean. Группа авторов
Чтение книги онлайн.
Читать онлайн книгу Plastics and the Ocean - Группа авторов страница 22
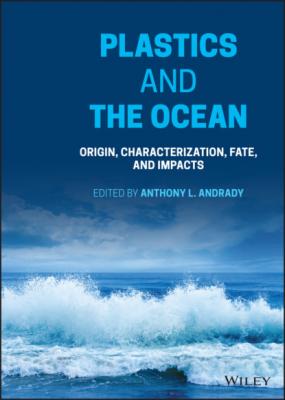
Two major strategies that conserve valuable fossil‐fuel resources and reduce the embedded environmental impacts are worth emphasizing: (i) extending the service life of plastic products; and (ii) increasing the use of bio‐based plastics, reducing the dependence on fossil fuel in manufacture.
1.4.1 Recycling of Post‐Use Plastics
Material recycling saves the energy expended for producing consumer plastic goods because it is the resin production (extraction, refining, and polymerization into resin) step, as opposed to the processing step, that has the higher embodied energy, EE (Schyns and Shaver 2020). Especially with single‐use products such as plastic cups, straws, or bags, the item is used only for a short duration and the quality of the plastic resin is barely affected by use. Being thermoplastic materials, the post‐use products can be collected and re‐melted for use in another generation of products. With most of the EE and embodied impacts associated with the manufacturing phase avoided, the strategy yields significant advantages (see Table 1.2). As seen from the table, savings in energy and in carbon emissions associated with recycling are substantial for plastics generally met with in the marine environment.
Even the leading type of plastic to be recycled in the US, PET bottles, are only recycled at a rate of ~28% presently. Even with bottles, the common use of the recyclate (r‐PET) resin is to “downcycle” into other products ( products of lower market value relative to the original high‐value bottle.) Alternatively, the r‐PET may be mixed with virgin PET (or used as a layer in multi‐layer extrusions) in fabricating original high‐value products. For instance, PET resin from plastic soda bottles might be downycled into textile or carpet fiber (Leonas 2017) and then downcycled again (mixed with other plastics) into lower value “plastic lumber” (Wan et al. 2019). Downcycling, however, is not the only recycling option available, as illustrated by the bottle‐to‐bottle recycling of PET, consistent with the paradigm of “circular economy.” To be sustainable, however, the US plastic industry also must join the ranks of high‐recyclers of the Word such as Norway, Sweden and South Korea, to recycle much higher fractions of at least the post‐consumer PET and HDPE, and encourage the recycling of other plastic resins as well.
Table 1.2 Comparison of the embodied energy (GJ/kg) and carbon footprint (kgCO2‐e) for virgin and recycled resin.
Based on data from Ashby (2013).
Plastic | Embodied energy Virgin (GJ/kg) | Embodied energy Recycled (GJ/kg) | Carbon footprint Virgin (kgCO2‐e /kg) | Carbon footprint Recycled (kgCO2‐e /kg) |
---|---|---|---|---|
Polyethylene | 77–85 | 45–55 | 2.6–2.9 | 2.7–3.0 |
Polypropylene | 75–83 | 45–55 | 2.9–3.2 | 2.0–2.2 |
Polyester (PET) | 81–89 | 35–43 | 5.7–4.1 | 2.1–2.6 |
Polystyrene | 92–102 | 43–52 | 3.6–4.0 | 2.6–3.1 |
Poly(vinyl chloride) | 56–62 | 32–40 | 2.4–2.6 | 1.9–2.4 |
Nylon 6 | 116–129 | 38–47 | 7.6–8.3 | 2.3–2.8 |
Poly(lactic acid) | 49–54 | 3.4–3.8 | 3.4–3.8 | 2.9–2.4 |
Low recycling rates in the US and elsewhere often result from the lack of readily‐accessible recycling infrastructure at the local level and the minimal market cost differential between virgin and recycled resin. Also, it is not always practical to mechanically recycle a mixed stream of plastic waste. For example, producing high‐quality recyclate is simply not possible with blends or multi‐layer laminated plastic products (Kaiser et al. 2018) or with products that are rendered photodegradable or enhanced biodegradable (a 0.5 wt% of biodegradable PLA contaminating a PET recycling stream discolors the rPET) (Alaerts et al. 2018). Some of waste plastics, however, may be amenable to recycling into chemical feedstock and energy via either pyrolysis or incineration. Recycling of waste fishing gear into plastic resin pellets, and their conversion to energy by incineration, are practiced at a small scale in the US, but much progress needs to be made.
Recycling does involve significant costs for collection, sorting, classifying, and cleaning of waste plastics, and there are incidental losses of material during the process that preclude a 100% mass recovery as the recyclate. Even with such losses, the savings in energy are substantial. There are, however, embedded impacts associated with the recycling process as well, and LCA must be carried out to make sure that most of what is saved in energy is not negated by increased environmental impacts. Also, each time a resin is recycled its mechanical characteristics detriorate; with PET, 3–5 repeated recycling results in ~50% decrease in tensile strength and extensibility of the resin (La Mantia and Vinci 1994).
1.4.2 Using Bio‐Based Feedstocks for Plastics.
Plastics can be classified based on the feedstock used in their synthesis, into those that are fossil fuel‐based and those derived from biological resources. Conventional plastics are mostly synthesized using feedstock derived from fossil fuel while the second category of plastics is synthesized using plant biomass as feedstock, and ISO, as well as the ASTM, refer to these as “bio‐based” plastics. The carbon in the plant biomass is derived from the present‐day atmosphere or the carbon cycle. However, it is important to recognize that “bio‐based” plastics defined in this manner include two distinct categories of plastics; those made from monomers derived from biomass and polymers synthesized by living organisms (or biopolymers) that are modified by man. Thus, three broad classes of biologically derives plastics might be identified.
1 Biopolymers that are synthesized by living organisms and exist as polymers in the biomass, including cellulose and poly (hydroxyl alkanoates) [PHAs]. These are extracted and used with no further chemical modification of the polymer.
2 Modified biopolymers where a biopolymer is extracted from biomass and chemically changed prior to use, as in the case of cellophane. Dissolving plant cellulose in carbon disulfide (in the xanthate process) or copper salt/ammonia (in viscose process), and re‐precipitating the solution in dilute acid yields cellophane and rayon. The material is still plant‐derived cellulose but with