Plastics and the Ocean. Группа авторов
Чтение книги онлайн.
Читать онлайн книгу Plastics and the Ocean - Группа авторов страница 19
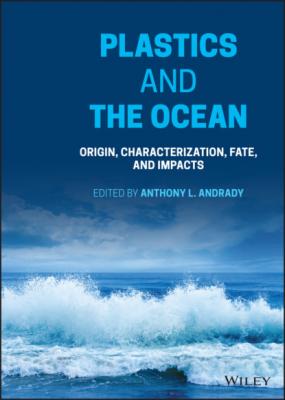
5 Introduction of alien species to new ecosystems by “rafters” or foulant species on the surface of floating plastic debris (Kiessling et al. 2015; Rech et al. 2018).
6 Possible development of antibiotic‐ and metal‐resistant microorganisms on foulant layers on plastics that have sorbed antibiotics through exposure to wastewater (Yang et al. 2019).
7 Interference with the operation of seagoing vessels by derelict fishing gear (Hong et al. 2017).
8 Potential contamination of fish and seafood leading to the increased human intake of MPs and NPs (Cox et al. 2019; see Chapter 13).
Of these problems, their ingestion by organisms, especially seafood species, is widely discussed because of the potential threat it poses to human health, in addition to the ocean ecosystem.
1.2.2 Microplastics in the Ocean
While the entanglement hazard of plastic macro‐debris such as derelict net fragments or six‐pack rings on large marine animals is easier to observe, it is the small‐sized debris not discernible visually that will have the more significant impact as they can interact with a much wider range of marine species. Particularly significant are fragments of plastics (<5 mm to 250 μm) generally referred to as “microplastics” (MPs) (Arthur et al. 2009). The term, however, is a misnomer as reports of “microplastics” in the ocean invariably include fragments with dimensions that are orders of magnitude larger than a micron. MPs constitute a very small weight fraction of the plastic debris in the ocean but are predominant in terms of their numerical abundance. It is their abundance rather than their mass per unit volume of water/sediment, that is directly related to potential threats to marine life.
There is obvious merit in subdividing the size range encompassed by microplastics into several narrow subranges, for instance, as in the classification proposed by the GESAMP (2016). Consistent with the nomenclature used by some researchers (Andrady 2011; Lusher 2015; Nelms et al. 2019), it is reasonable to specify the narrower size range of 1 mm to 1 μm as “true” microplastics (with those smaller, as nano plastics (NPs), and larger as mesoplastics). MPs also occur in different geometries, including microfibers, plastic fragments, films, pieces of foam (such as PS foam and polyurethane), and virgin resin pellets that are believed to “leak” into the environment from plastic processing operations as well as during the transportation of resin (Karlsson et al. 2018). Geometry‐specific physiological impacts are possible with the ingestion of MPs, especially the NPs (Danopoulos et al. 2021). Nanoplastics derived from MPs or larger debris are an especially important category because their size allows a very large range of marine animals to ingest these.
Secondary MPs derived from mesoplastics by fragmentation may degrade further yielding NPs (Andrady 2017; Chapter 8). Some data suggests the NPs that are extensively weathered contribute to the pool of dissolved organic carbon (DOC in seawater (Zhu et al. 2020; Romera‐Castillo et al. 2018).
Plastic micro‐debris is further categorized as primary and secondary, based on their origin.
1 Primary MPs are intentionally manufactured in that size scale for use in a specific application; and
2 Secondary MPs result from the fragmentation of plastic macro litter in the ocean environment, often assisted by their weathering degradation as discussed in Chapter 8 (Andrady 2017; Barnes et al. 2009). Though fragmentation may also occur with virgin material, plastics that have undergone extensive weathering under exposure to solar UVR, and therefore weakened or embrittled, tend to fragment far more easily in the environment (see Chapter 8.)
Examples of primary MPs include manufactured microbeads used as exfoliant additives in personal care products such as facial creams and toothpaste (Fendall and Sewell 2009). These microbeads typically have a size range of 164–327 μm (Napper et al. 2015) and are predominantly made of polyethylene (Gouin et al. 2015); therefore, at least initially, float in seawater (Van Cauwenberghe et al. 2015). They are accessible for ingestion by a range of smaller marine organisms that populate the photic upper layer in the ocean. However, the use of microbeads in rinse‐off type cosmetics has been phased out in 2019–2020 in both the US and the EU, preventing 1500 tons per year of these from entering the aquatic environment (Sun et al. 2020). But noncosmetic uses of microbeads that include plastic‐blasting media, textile printing, and biomedical applications (Leslie 2014) continue worldwide.
Microfibers (MF) in the environment can originate from either textile manufacturing or fabric laundering (Cesa et al. 2020), especially when using top‐loading washing machines. A single wash cycle of a garment (a fleece jacket) in detergent‐free water in a household washing machine releases about 0.3% of its weight as MFs (Hartline et al. 2016) while studies have found 1079 to 106 fibers per kg fabric in five repeated washes to be released into wastewater (Zambrano et al. 2019; Falco et al. 2019). Furthermore, textile fibers such as nylon or polyester can undergo fragmentation consequent to weathering on exposure to solar UVR (Sait et al. 2021). These microfibers can be micro‐ (<100 μm) as well as nanoscale (<100 nm) (Mintenig et al. 2017) and pose a similar threat to marine biota as MPs (Henry et al. 2019). How much fiber exists as debris in the ocean, is difficult to assess, especially because textile fibers are denser than seawater. A recent estimate places it at ~0.2 MMT released annually; as with MPs, the ecological significance of MFs does not scale with the tonnage but with the particle abundance of these in seawater. However, fibers found in seawater collected from six ocean basins turned out to be predominantly (79.5%) cellulosic with synthetic fibers constituting only 8.2% of the microfibers (Cesa et al. 2017). This observation is inconsistent with >50% volume production being synthetic recalcitrant fibers; but the denser synthetics fibers are more likely to be in the sediment. The high percentage of inherently biodegradable cellulosic fiber in the ocean is yet to be explained.
A summary compilation of available datasets on the abundance of MPs in the ocean (particles/m3) is shown in Figure 1.6 for particles in the size scale of 100–5000 μm. But floating‐stock assessments are invariably underestimates because of the size limit imposed by the mesh size of the sampling nets and the collecting effectiveness of different sampling gear (Zheng et al. 2021). Lindeque et al. (2020) found the MPs concentrations to be an order of magnitude larger when sampling with a 10 μm mesh as opposed to a 500 μm mesh net. For instance, N. Atlantic surface water sampled at 23 sites using a 10 μm mesh net (Enders et al. 2015) yielded MPs in the size range of 10–150 μm,while using a 200 μm mesh net to collect samples at 141 sites during a global circumnavigation cruise (Cozar et al. 2014), yielded MPs in the size range of 0.4–15 mm. Even taking into account the differences in location and identification, these samples likely belong to statistically different populations. Regardless of such drawbacks, however, all efforts at quantifying sea‐surface plastics will still capture only ~1% of the total influx of plastics into the ocean as most would have sunk into the sediment.
Figure 1.6 Density (log particles/m3) of MPs (100–5000 μm) in the different oceans. Horizontal line: median; box boundaries: 25th and 75th percentiles; bars: range of observed values; dots and asterisks: potential outliers.
Source: Courtesy Beiras