Plastics and the Ocean. Группа авторов
Чтение книги онлайн.
Читать онлайн книгу Plastics and the Ocean - Группа авторов страница 45
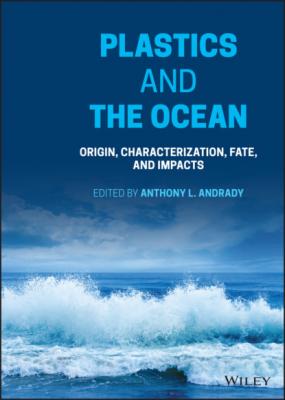
Note: Sample sizes are 1, 4, 5, 50, and 50 from left to right, respectively. Dotted bars indicate all fish were below the shown limit of quantification. Error bars indicate standard deviation or standard error provided in respective paper.
For human dietary intake studies, the tissue that is most frequently consumed (e.g. fillets) was analyzed. In trophic transfer studies, the whole fish was analyzed including the gastrointestinal tract which may contain ingested plastics. On fewer occasions, fish liver was analyzed and compared to muscle tissue for APs (Lye et al. 1999), bisphenols (Barboza et al. 2020), and also to gill and kidney for phthalates (Adeogun et al. 2015). Seabird eggs offer lipid‐rich samples suitable for long‐term monitoring programs (Law et al. 2014), and have been analyzed for additives beyond BFRs (Lundebye et al. 2010) and compared to liver concentrations (Hallanger et al. 2015). Likewise, marine mammal blubber is commonly analyzed for BFRs, because of their accumulation in fatty tissues. Blubber and plasma have been analyzed for benzotriazole UV stabilizers and for substituted diphenylamine antioxidants (Lu et al. 2016; Nakata et al. 2010). Phosphate‐based additive concentrations have been compared among the blubber, brain, kidney, liver, muscle, and plasma of marine mammals (Hallanger et al. 2015; Sala et al. 2019). Phthalate concentrations have been compared among sea turtle fat, gonads, liver, and muscle (Savoca et al. 2018), detected in seabird preen oil (Provencher et al. 2020) and in marine mammal liver (Rian et al. 2020). Since phthalates are quickly metabolized and eliminated from the body (Staples et al. 1997), some studies have targeted phthalate metabolites, instead of or in addition to the parent compound, in fish muscle (Fossi et al. 2014). Also, marine mammal blubber (Fossi et al. 2012), skin (Fossi et al. 2016), and urine (Hart et al. 2018, 2020) have also been studied in this regard. Urine concentrations of phthalates are the most widely used approach in human biomonitoring studies (Wang et al. 2019).
Certain additives can biomagnify in food webs, while others, like plastics themselves, do not (Covernton et al. 2021; Koelmans et al. 2016; Tomy et al. 2008). From our database, 12 studies investigated biomagnification of additives in marine food webs (see Box 1.2 in Chapter 1 for a discussion of biomagnification). Phthalates did not biomagnify significantly (Mackintosh et al. 2004; Morin 2003). Certain congeners of PBDEs biomagnified in several marine food webs (Brandsma et al. 2015; Mizukawa et al. 2009; Tomy et al. 2008), but conflicting results have been obtained for HBCDs (Brandsma et al. 2015; Tomy et al. 2008; Zhang et al. 2018c). One study provided evidence of TBBPA biomagnification (Li et al. 2021). Phosphate‐based FRs also did not biomagnify in three marine food webs but tentatively did in a benthic food web (Brandsma et al. 2015; Garcia‐Garin et al. 2020; Hallanger et al. 2015). There is some tentative evidence of biomagnification of benzotriazole UV stabilizers (Nakata et al. 2010; Peng et al. 2017). BPA most likely does not biomagnify (Corrales et al. 2015; Gu et al. 2016), and there is only weak correlative evidence for the biomagnification of 4‐t‐OP and 4‐n‐NP (Gu et al. 2016). We are unaware of trophic transfer studies for additive classes such as other antioxidants, heat stabilizers, fillers, IMs, colorants, or lubricants.
2.6 Toxicity of Additives
The toxicity of plastic additives is quite variable given the diversity of their chemical classes. Determining the dose that kills 50% of a test animal (LD50), such as Daphnia magna in 48‐hour exposures, is a basic standard aquatic toxicity test that allows for simple comparisons of toxicity across compounds. The range of LD50 values for plastic additives exemplifies this diversity (Table 2.5). Acutely toxic additives (e.g., LD50 values <1 mg/L) include lower brominated PBDEs, APs, some phthalates, such as DEHP, Basic Red 51 azo dye, cadmium, copper, and zinc. In contrast, other additives, considered not harmful because their LD50 values are >10 mg/L, include 2,2‐bis(bromomethyl)‐1,3‐propanediol (BBMP), TCEP, and DEP. The antioxidant, Irganox 1010 has a high LD50 for D. magna (86 mg/L, Table 2.5) and other animals; therefore, it is allowed in food‐contact plastic packaging (USFDA 2019). Lower toxicity provides some justification for replacing conventional additives with newer replacements. Even so, many replacements still exhibit some level of toxicity, some even at similar concentrations than the original additive, and should be more thoroughly studied (Behl et al. 2016; Luo et al. 2021).
The toxicity of many plastic additives has been reviewed previously (Table 2.6). Endocrine disruption is a prominent toxicological mechanism noted in the literature for many plastic additives. Endocrine‐disrupting chemicals (EDCs) are so‐called because they disturb any step in the complex feedback systems of hormones that regulate reproduction, growth, metabolism, and many other biological functions. Mechanisms of toxic action include interfering in the synthesis, activity, or elimination of hormones or their receptors. Phthalates, FRs, antioxidants, monomers, and metal‐based additives are known as EDCs, disrupting a range of different hormonal systems (Table 2.7). The main concern over EDCs is that they act at concentrations much lower than lethal concentrations and results in sublethal effects, such as reduced reproduction or slower growth, both of which could significantly harm populations or communities of marine organisms. For example, thyroid disruption and neurobehavioral effects were observed in crucian carp (Carassius auratus) at concentrations of HBCDs 10–100 times higher than environmental concentrations, leaving only a small margin of safety for wild fish populations (Dong et al. 2018).
Additives can also exert neurological, carcinogenic, developmental, immunotoxic, and organ toxicities. DEHP, for instance, is an animal and human carcinogen (Campanale et al. 2020). Toxicity tests of organophosphorous flame retardants (OPFRs) with mammals, birds, and fish resulted in neurotoxicity, oxidative stress, altered metabolic processes, developmental toxicity, and effects on the liver, kidney, and other organs (Du et al. 2019). Hindered phenolic antioxidants, including 2,6‐di‐tert‐butyl‐4‐methylphenol (BHT) and butylated hydroxyanisole (BHA) and their metabolites, exhibit diverse toxicities, including endocrine disruption, kidney and liver effects, genotoxicity, tumor promotion or enhancement, reproductive effects, and lipid disruption (Liu and Maybury 2020). The diverse toxic effects of priority metals, such as As, Cd, Cr, Pb, and Hg, to multiple organs are well known (Liu et al. 2008).
Table 2.6 Examples of review articles discussing the toxicities of plastic additives.
Reference | Chemical class | Toxicological effect | Organismal focus for toxicology |
---|---|---|---|
Hermabessiere et al. (2017) | Multiple | Multiple | Marine |
Liu et al. (2020) | Multiple | Ecotox proteomics | Aquatic |
Pérez‐Albaladejo et al. (2020) | Multiple | Oxidative stress | Human and Aquatic |
Oehlmann et al. (2009) | Phthalates, bisphenol A | Multiple | Aquatic and terrestrial |
Staples et al. (1997) | Phthalates | Acute and Chronic | Aquatic |
|