Acoustic and Vibrational Enhanced Oil Recovery. George V. Chilingar
Чтение книги онлайн.
Читать онлайн книгу Acoustic and Vibrational Enhanced Oil Recovery - George V. Chilingar страница 12
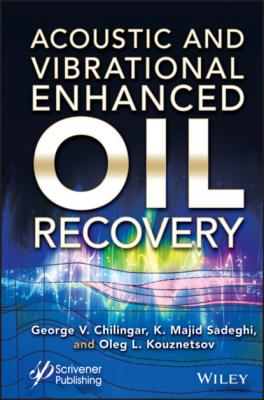
In case where imposed electric potential gradient, E, is in the same direction as the pressure drop (Δp), the total flow rate (qt) upon application of D.C. current is as follows:
where k is the hydrodynamic permeability;
Figure 1.3 Schematic diagram of EEOR (Electrical Enhanced Oil Recovery) electrode arrangement in oil fields as used by Wittle et al. (2008) [38] and (2011) [39], Ambah et al. (1965) [1], and Titus et al. (1985) [35].
Equation (1.1) can be presented in a dimensionless form by normalizing the flow rates and, thus, eliminating µ, A, and L terms (Chilingar et al., 1970 [10]):
(1.2)
and
(1.3)
where qi is the initial hydrodynamic stabilized flow rate:
(1.4)
Thus, the possible existence of strong D.C. current in the rocks during the geologic past could have facilitated the movement of fluids (Ambah et al., 1965 [1]).
1.1.3 Earth Tides
In the classic book on wettability, Donaldson and Alam (2013) [13] pointed out that Earth tides activate the motion of fluids by continued expansion and contraction as simple harmonic oscillation resulting from diurnal tides. This effect was much greater earlier in geologic history because the moon was much closer to the Earth. Currently, the moon is moving away from the Earth at ≈3 cm/yr. Thus, the Earth tides were more rapid and energetic, resulting in greater dilation and the compression of rocks. According to Donaldson and Alam, in a 10-m-thick reservoir, with a radius of 100 m, an elastic change of porosity of 0.1% will result in an oscillatory motion of 294 bbl of fluid. Such motions could reduce the oil saturation to zero over geologic time, leaving only a trace amount of oil behind.
1.1.4 Compaction
In order to investigate some problems involved in the primary migration of oil, Aoyagi et al. (1985) [3] compacted Na-monmorillonite clay mixed with seawater and crude oil for 25 days under a pressure of 1,000 kg/cm2 and a temperature of 60°C. The proportion of oil in the expelled liquid increased with time. The porosity of the compacted sample decreased from 81% to ≈26%. These authors concluded that the primary migration of oil from source rocks to reservoir rocks occurred chiefly during the late compaction state (ϕ = 10%−30%). Considering the fact that tectonic activity during the primary migration of oil was intense, the oil was simply squeezed out (expelled) from the source rock.
1.1.5 Migration in a Gaseous Form
Kapelyushnikov (1954) [17], Gerber and Dvali (1961) [15], Zaks (1952 [40], 1955 [41]), Zhuze and Yushkevich (1959) [42], and Chilingar and Adamson (1964) [9] studied the possibility of oil migration in a gaseous form. Based on some experimental work, Chilingar and Adamson (1964) [9] concluded that some migration of petroleum could have occurred in a gaseous form in the geologic past at high temperatures.
It is important to remember that with increasing polarity of oil, the relative permeability to oil increases and that to water decreases (water cut decreases) (Sinnokrot and Chilingar, 1961 [32]).
Gerber and Dvali (1961) [15] subjected to extraction four samples of rocks with CO2 at pressures ranging from 100 to 400 kg/cm2 and temperatures of 40°C to 74°−90°C. Their findings can be summarized as follows:
1 The bitumens dispersed in rocks and, having composition related to crude oil, can dissolve in compressed gases and migrate together with them.
2 At 200 to 400 kg/cm2 pressure and temperature of 40°C, in dynamic conditions, CO2 can extract not only oils and tars but also asphaltenes and porphyrins from bituminous shales of Ukhta (Russia).
3 The kerosene and the main oil fraction of bitumens can be extracted from the rocks at pressures of 100 to 200 kg/cm2 and temperature of 40°C.
4 Mobile, syngenetic bitumens in the source rocks can dissolve just as easily in the compressed CO2 gas as does petroleum from oil-saturated rocks.
Kapelyushnikov (1954) [17], who studied the P-V-T relationship of oil, gas, and water at pressures up to 500 atmospheres and temperatures up to 100°C, concluded that gaseous oil/gas mixtures move toward the low-pressure areas, dropping the tars and asphaltenes first, followed by medium and lighter components. He also found that at critical pressures, the water and salts present in the oil-bearing strata are also transported together with the gas toward the low pressure areas.
1.2 Seismic Vibration Techniques
The use of various seismic vibration techniques in Russia resulted in incremental oil production of millions of tons. There are several seismic vibration techniques for transmitting energy into the reservoir, with oscillations over long distance. The area of productive reservoir around the well-being stimulated may be as great as 12 km2. The number of wells simultaneously subjected to the treatment can range up to 50 (depending on the well spacing).
The technique for increasing the oil yield of high water cut and low oil production reservoirs consists of subjecting the reservoir area to cyclic low-frequency (5 to 90 HZ) elastic oscillations within the frequency range corresponding to the reservoir resonance (Kouznetsov et al., 1998 [18]). The results obtained for during seven years of using this seismic vibration technique in different areas in Russia, on the average, annual oil production increased by more than 60%. The duration of the seismic vibration effect was 6 to 18 months and sometimes longer. The vertical reservoir sweep increased by 35%. In some cases, the wells that were producing by artificial lift (sucker rod pumps) retuned to natural flow with almost a tenfold rate increase. The effect was evaluated not only by an increase in the total production rate but also by a decrease in the waste cut. In some wells, the water cut decreased by 30% to 40% (Kouznetsov et al., 1998 [18]; Simkin and Surguchev, 1991 [31]).