Microgrid Technologies. Группа авторов
Чтение книги онлайн.
Читать онлайн книгу Microgrid Technologies - Группа авторов страница 22
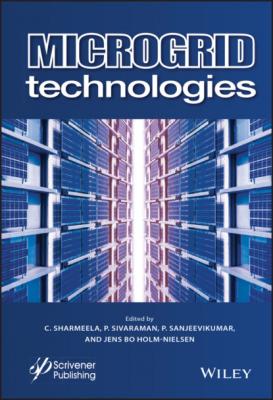
2.2.1.2 Mathematical Modeling of PAFC Fuel Cell
The output voltage of fuel cell is given by Nernst equation as [8–11]:
(2.1)
Table 2.1 Fuel cell resource comparison (courtesy: DOE, US Government).
Figure 2.2 PAFC characteristics.
Figure 2.3 PAFC average cell voltage vs current density.
Where:
Vdc = Fuel cell output voltage (Volt)
E = Thermodynamic potential of fuel cell (Volt) which is expressed by:
(2.2)
E0 = Potential of unit cavity in fuel cell = 1.229 V
PH2, PO2 & PH2O = Particle pressure of Hydrogen, Oxygen and vapor (atm)
T = Cell temperature (K)
R = Universal gas constant = 8.31441 J/mol-K
F = Faraday constant = 94,685 C/mol
n = Number of electrons participating in the reaction
V0 = Ohmic voltage drop (Volt) which is given by:
(2.3)
I = Cell current (Amp)
Rint = Internal resistance between electrodes which is given by:
l = Length between two electrodes (Meter)
Va = Summation of activation voltage drop and concentration voltage drop (Volt) which is expressed by:
(2.4)
α = Electron transfer co-efficient
i = Current density (A/m2)
i0 = Exchange current density (A/m2)
iL = Limiting current density (A/m2)
є = Electrical permittivity of electrolyte
A = Effective surface area between electrodes and electrolyte (sq. meter). It is very large due to corrugated porous surface of the electrodes.
l = Distance between two layers of electrodes. It is very small (nanometer).
VC = Voltage across the Capacitor ‘C’. It is given by:
(2.5)
Figure 2.4 PAFC equivalent circuit.
There are three operating regions for this fuel cell. The first region is activation region where the voltage drop is due to the slowness of the chemical reactions at electrode. It is considered as Ra. The second region represents the ohmic losses due to the internal resistance of the fuel cell. It is represented by Ro. The third region represents the change in concentration of reactants as the fuel is used. It is represented by Rc. By considering these three operating regions of fuel cell, an equivalent DC circuit model is obtained as shown in Figure 2.4.
2.3 Power Flow Management in Microgrid
The transfer capacity of the existing transmission lines is an important operational constraint on interconnected A.C. transmission network. To improve this capacity of the transmission line, one has to use the FACTS controllers. These controllers are known for their applications in improving power transfer capacity and power flow control using the existing infrastructure of a transmission utility as well as improving transient stability. In addition to these controls, FACTS controllers advantageously employed for transient stability improvement, power oscillation damping and voltage stability. With the FACTS controller, the transmission line capacity can be improved by 40 to 50% as compared to conventional mechanically-driven devices. Lower maintenance required for FACTS controllers improves effi-ciency in operation [1].
The FACTS controllers connected to the transmission line have following basic types depending on connection:
1 Series controllers:It has variable impedance and has a function to inject series voltage.
2 Shunt controllers:It has variable impedance and has a function to inject current in the system.
3 Combined series–series controller:It has separate but coordinated unified (common DC bus) controllers and can compensate reactive power as well as interline transfer active power.
4 Combined series–shunt controller:It can inject voltage and current and has unified and coordinated real power exchange between series and shunt controllers.
The UPFC, as shown in Figure 2.5, invented in 1991, is a real-time multi-functional dynamic compensator for the AC transmission line. It can control active and reactive power as well as voltage and VAR compensation. It also can improve the power quality of the associated system.