Flexible Supercapacitors. Группа авторов
Чтение книги онлайн.
Читать онлайн книгу Flexible Supercapacitors - Группа авторов страница 17
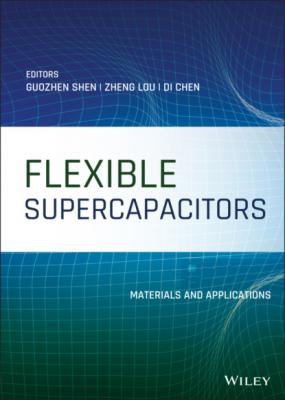
Source: Reproduced with permission [36]. © 2013, Wiley‐VCH.
2D planar SCs, the individual elements are:
Source: Reproduced with permission [37]. © 2013, Wiley‐VCH. Reproduced with permission [38]. © 2013, American Chemical Society. Reproduced with permission [39]. © 2017, Wiley‐VCH.
3D SCs,
Source: Reproduced with permission [40]. © 2016, American Chemical Society.
Multifunctional: self‐healable SCs,
Source: Reproduced with permission [31]. © 2015, Nature Publishing Group.
Compressible SCs,
Source: Reproduced with permission [41]. © 2015, WILEY‐VCH.
Integrated system,
Source: Reproduced with permission [101]. © 2018, Elsevier Ltd.
By design of different structure, the electrochemical performance of fiber SCs can be improved. For example, Peng's group [51] made a comparative study to analyze electrochemical performance of coaxial and twisted SCs, in detail, carbon nanotube (CNT) sheet was scrolled on the another CNT sheets with a gel electrolyte coat to fabricate coaxial devices and two CNT sheets fiber electrodes were intertwined to obtain twisted SCs, the electrochemical tests showed that coaxial SCs exhibit larger specific capacitance of 59 F/g than that of twisted SCs (4.5 F g−1) because the distance between positive and negative electrodes of the coaxial SCs is more closer and the utilization of materials is more effective compared to the twisted one. In order to understand how the configuration effect on the electrochemical performance of fiber shaped SCs, our group built a theoretical model using ANSYS Maxwell software [52]. The simulated parameters used in simulation system were particularly organized to ensure the unity of volume, superficial area, materials, relative permittivity, and positive/negative voltage between coaxial and twisted geometries. At first, two electrode models were carried out with the same electrode material and electrolyte parameters, the voltage of electrode was set from −0.4 V to 0.4 V. The analysis results on electric field under the same conductions were displayed, as shown in Figure 2.2. Figure 2.2a and b depicted the schematic diagram of the coaxial and twisted fiber SCs used in the theoretical model. Figure 2.2c and d displayed the corresponding electrostatic potential distribution around the two fiber SCs. Compared to the scattered, asymmetrical, and sigmoid potential distribution of the twisted devices, the potential distribution of coaxial SCs is more homogeneous, leading to the better electric charge‐transfer effect and lower contact resistance. Figure 2.2e and f showed the energy distribution simulation results of the twisted and coaxial devices.
Figure 2.2 Structure and voltage, energy distribution of 1D fiber SCs: twisted and coaxial. (a, b) Schematic diagram of the coaxial and twisted fiber SCs. (c–f) The voltage distribution and energy distribution of the two structures simulated by ANSYS Maxwell software.
Source: Reproduced with permission [52]. © 2018, Wiley‐VCH.
According to the following equation, the energy densities (E) and capacitance (C) can be calculated:
(2.1)
The Eq. (2.2) was obtained as:
Then capacitance matrix is derived from Eq. (2.2) as:
(2.3)
Where Q represents the quantity of electric charge, V stands for voltage.
A capacitance ratio of 5.14 : 1 was obtained between the two structures, confirming and explaining the better electrochemical performances of the coaxial SCs coming from the shorter distance between positive and negative electrode, more uniform potential distribution and better conductivity. But the fabrication process of the coaxial SCs is much more complicated in comparison with twisted devices. These simulation results provide a theoretical support to the design of the fiber SCs from the views of the device configuration, which can be applied and extended to other filed that refers to the devices structures.
2.2.1.1 Fabrication of Stretchable Parallel SCs
The fabrication of stretchable, fiber‐shaped SCs with parallel structure is relatively easy. There are two typical ways to realize stretchability: one is by packaging the devices into stretchable substrate, another is by employing stretchable substrate or designing special structure, like screw spring, serpentine, etc. to implement it [53–55]. Figure 2.3a concretely displayed the first group of stretchable SCs by embedding the whole devices into pre‐strained stretchable substrate [56]. The CNT fibers, which were prepared by drawing and twisting CNT strips out of a vertically aligned CNTs array, were used as the wire‐shaped current collector. The highly aligned inner structures can provide both excellent mechanical properties and high electrical conductivity. Afterwards,(KMnO4) was used. Then, the two MnO2 coated CNT fibers were placed parallelly into pre‐strained stretchable substrate, after releasing, the stretchable fiber SCs were obtained with serpentine structures. Owing to the excellent electrochemical performance of MnO2 nanomaterials, the outstanding electrical conductivity of CNT fibers as well as the pre‐straining method, the fabricated fiber SCs showed a specific volumetric capacitance of ≈409.4 F cm−3 at 0.75 A cm−3at 40% of tensile strength. Figure 2.3b exhibited the cyclic voltammetry (CV) curves of the stretchable devices under stretching and folding, nearly invariable CV curves under different deformation state demonstrated the successful assembly of the stretchable fiber SCs. Although this method achieved the stretchability, the SC device itself did not live up to the conception of stretchability.