Isotopic Constraints on Earth System Processes. Группа авторов
Чтение книги онлайн.
Читать онлайн книгу Isotopic Constraints on Earth System Processes - Группа авторов страница 28
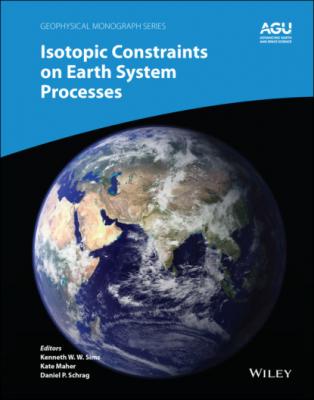
The present report limited itself to kinetic isotope effects in high‐temperature silicate systems. The interested reader will find a discussion of a large number of works reporting kinetic isotope fractionations in aqueous solutions in Watkins et al. (2017).
REFERENCES
1 Beck P., Chaussidon, M., Barrat, J. A., Gillet, Ph., & Bohn, M. (2006). Diffusion induced Li isotopic fractionation during the cooling of magmatic rocks: The case of pyroxene phenocrysts from nakhlite meteorites. Geochimica et Cosmochimica Acta, 70, 4813–4825. doi: 10.1016/j.gca.2006.07.025
2 Bourg, I. C., Richter, F. M., Christensen, J. N., & Sposito, G. (2010). Isotopic mass‐dependence of metal cation diffusion coefficients in liquid water. Geochimica et Cosmochimica Acta, 74, 2249–2256. https://doi.org/10.1016/j.gca.2010.01.024
3 Bowen, N. L. (1921). Diffusion in silicate melts. Journal of Geology, 29, 295–317. https://doi.org/10.1086/622784
4 Chapman, S., & Dootson, M. A. (1917). A note on thermal diffusion. The London, Edinburgh, and Dublin Philosophical Magazine and Journal of Science, 33, 248–253. https://doi.org/10.1080/14786440308635635
5 Chopra, R., Richter, F. M., & Watson, E. B. (2012). Isotope fractionation by chemical diffusion in natural settings and in their laboratory analogues. Geochimica et Cosmochimica Acta, 88, 1–18. https://doi.org/10.1016/j.gca.2012.03.039
6 Clayton, R. N., Hinton, R. W., & Davis A. M. (1988). Isotopic variations in the rock‐forming elements in meteorites. Philosophical Transactions of the Royal Society of London A, 325, 483–501. https://doi.org/10.1098/rsta.1988.0062
7 Cooper, A. R. (1968). The use and limitations of the concept of an effective binary diffusion coefficient for multicomponent diffusion. In: J. B. Wachtman and A. D. Franklin (eds.), Mass Transport in Oxides, NBS Special Publication 296, 79–84.
8 Davis, A. M., Hashimoto, A., Clayton, R. N., & Mayeda, T. K. (1990). Isotope mass fractionation during evaporation of Mg2SiO4. Nature, 347, 655–658. https://doi.org/10.1038/347655a0
9 de Groot, S. R., & Mazur, P. (1962). Non‐Equilibrium Thermodynamics. Dover.
10 Dohmen, R., Kasemann, S. A., Coogan, L. A., & Chakraborty, S. (2010). Diffusion of Li in olivine. Part 1: Experimental observations and a multiple species diffusion model. Geochimica et Cosmochimica Acta, 74, 274–292. https://doi.org/10.1016/j.gca.2009.10.016
11 Enskog, D. (1917). Kinetische Theorie der Vorgaenge in maessig verduennten Gasen. I. Allgemeiner Teil, Uppsala.
12 Fedkin, A. V., Grossman, L., Humayun, M., Simon, S. B., & Campbell, A. J. (2015). Condensates from vapor made by impacts between metal‐, silicate‐rich bodies: Comparison with metal and chondrules in CB chondrites. Geochimica et Cosmochimica Acta, 164, 236–261. https://doi.org/10.1016/j.gca.2015.05.022
13 Galy, A., Yoffe, O., Janney, P. E., Williams, R. W., Cloquet, C., Alard, O., et al. (2003). Magnesium isotope heterogeneity of the isotopic standard SRM980 and new reference materials for magnesium‐isotope ratio measurements. Journal of Analytical Atomic Spectrometry, 18, 1352–1356. https://doi.org/10.1039/B309273A
14 Goel, G., Zhang, L., Lacks, D. J., & Van Orman, J. A. (2012). Isotope fractionation by diffusion in silicate melts: Insights from molecular dynamics simulations. Geochimica et Cosmochimica Acta, 93, 205–213. https://doi.org/10.1016/j.gca.2012.07.008
15 Grossman, L., Ebel, D. S., Simon, S. B., Davis, A. M., Richter, F. M., & Parsad, N. M. (2000). Major element chemical and isotopic composition of refractory inclusions in C3 chondrites: The separate roles of condensation and evaporation. Geochimica et Cosmochimica Acta, 64, 2879–2894. https://doi.org/10.1016/S0016‐7037(00)00396‐3
16 Guo, C., & Zhang, Y. (2018). Multicomponent diffusion in basaltic melts at 1350°C. Geochimica et Cosmochimica Acta, 228, 190–204. https://doi.org/10.1016/j.gca.2018.02.043
17 Hashimoto, A. (1999). Chemical and isotopic fractionations in the primordial nebula. Planet. People, 4, 266–282.
18 Holycross, M. E., Watson, E. B., Richter, F. M., & Villeneuve, J. (2018). Diffusive fractionation of Li isotopes in wet, highly silicic melts. Geochemical Perspectives Letters, 6, 39–42. doi: 10.7185/geochemlet.1807
19 Jeffcoate, A. B., Elliott, T., Kasemann, S. A., Ionov, D., Cooper, K., & Brooker, R. (2007). Li isotope fractionation in peridotites and mafic melts. Geochimica et Cosmochimica Acta, 71, 202–218. https://doi.org/10.1016/j.gca.2006.06.1611
20 Knight, K. B., Kita, N. T., Mendybaev, R. A., Richter, F. M., Davis, A. M., & Valley, J. W. (2009). Si isotope fractionation of CAI‐like vacuum evaporation residues. Geochimica et Cosmochimica Acta, 73, 6390–6401. https://doi.org/10.1016/j.gca.2009.07.008
21 Kyser, T. K., Lesher, C. E., & Walker, D. (1998). The effects of liquid immiscibility and thermal diffusion on oxygen isotopes in silicate liquids. Contributions to Mineralogy and Petrology, 133, 373–381. https://doi.org/10.1007/s004100050459
22 Lesher, C. E., & Walker, D. (1986). Solution properties of silicate liquids from thermal diffusion experiments. Geochimica et Cosmochimica Acta, 50, 1397–1411. https://doi.org/10.1016/0016‐7037(86)90313‐3
23 Liang, Y., Richter, F. M., Davis, A.M., & Watson, E. B. (1996a). Diffusion in silicate melts: I. Self‐diffusion in CaO‐A2O3‐Si02 at 1500°C and 1 GPa. Geochimica et Cosmochimica Acta, 60, 4353–4367. https://doi.org/10.1016/S0016‐7037(96)00288‐8
24 Liang,