Molecular Mechanisms of Photosynthesis. Robert E. Blankenship
Чтение книги онлайн.
Читать онлайн книгу Molecular Mechanisms of Photosynthesis - Robert E. Blankenship страница 23
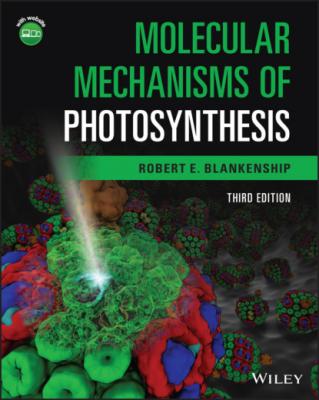
The information that the photosynthetic quotient is 1, and that the organic matter is a carbohydrate such as starch or sugar, allows us to write a minimally balanced equation for photosynthesis:
(3.2)
where (CH2O) is representative of a carbohydrate. One example of a carbohydrate is glucose, C6H12O6, which makes the overall balanced photosynthetic equation:
(3.3)
As we will see in Chapter 9, glucose is not the carbohydrate directly formed in photosynthesis, but it has almost the same energy content, so this is adequate for our present needs.
Julius Robert Mayer (1814–1878), a German physician and physicist who first enunciated the law of conservation of energy, proposed in 1845 that in photosynthesis light energy is converted to chemical energy, thus completing the formulation of the equation of photosynthesis.
3.7 Early mechanistic ideas of photosynthesis
When the overall reaction of photosynthesis had been established, attention turned to elucidating the details of the mechanism of the process. The early ideas in this regard were erroneous and much too simplistic. Richard Willstätter (1872–1942) and Arthur Stoll (1887–1971) proposed in 1918 that the product was actually formed directly as a molecular species, formaldehyde (CH2O), in a direct, concerted process involving chlorophyll, CO2, and H2O. This view was revived later by Otto Warburg (1883–1970) in support of his unorthodox formulation of photosynthesis, which we will discuss a little later. We now know that these mechanistic ideas, which were certainly reasonable at the time, are not valid, because there are literally dozens of intermediate states that have been identified, and the reduction in CO2 can be separated from the production of oxygen, and vice versa. The key to understanding in more detail how the mechanism of photosynthesis really works came from the analysis of simple photosynthetic organisms by van Niel and by Hill's experiments showing that CO2 reduction and O2 evolution can be decoupled.
3.7.1 Van Niel and the redox nature of photosynthesis
The cornerstone of our current understanding of photosynthesis is that it is a light‐induced reduction–oxidation (redox) chemical process. This principle was first clearly set forth in the 1930s by the Dutch microbiologist Cornelis van Niel (1897–1985), working at Stanford University. Van Niel carried out a series of experiments on the metabolic characteristics of non‐oxygen‐evolving (anoxygenic) photosynthetic bacteria (van Niel, 1941). These organisms contain bacteriochlorophylls, pigments related to, but distinct from, the chlorophylls contained in cyanobacteria, algae, and plants. They assimilate CO2 into organic matter, but do not produce molecular oxygen. In order for these bacteria to assimilate CO2, they must be supplied with a reducing compound. Many different compounds will suffice, most notably H2S, which is first oxidized to elemental sulfur and then further oxidized. In place of H2S, a variety of organic compounds can also be utilized, or even molecular hydrogen. Van Niel's seminal contribution was the recognition that these compounds could all be represented by the general formula H2A, and that the overall equation of photosynthesis could be reformulated in a more general way as follows:
(3.4)
The oxygen‐evolving form of photosynthesis can then be seen as a special case of this more general formulation, in which H2O is H2A and O2 is 2A. When presented in this manner, the redox nature of photosynthesis is much more obvious. In fact, it is a simple further step to separate the oxidation and reduction into two chemical equations, one for the oxidation and the other for the reduction:
This separation into oxidation and reduction reactions leads to a number of important predictions. First, it suggests that the two processes might possibly be physically or temporally separated. Indeed, this was found to be the case by Hill in his classic experiments on the use of artificial electron acceptors, which will be discussed in the next paragraph. A second prediction is that the oxygen produced by oxygen‐evolving photosynthesis comes from H2O and not from CO2. This is indeed the case, although it took many years for this fact to be established unequivocally, because of the rapid exchange of oxygen between CO2 and H2O via carbonic acid, H2CO3, which is constantly forming and breaking down. Van Niel correctly imagined that the oxidation and reduction reactions of Eqs. (3.5a) and (3.5b) were not the primary processes carried out by light, but were, rather, the result of a primary oxidant and reductant generated in the light, which went on to react with the substrates to form the products. However, some of van Niel's detailed ideas about the nature of the primary oxidant and reductant were incorrect, and he was overly rigid in insisting that all assimilated carbon had first to be oxidized all the way to CO2 before it could be subsequently reduced. Nevertheless, the truth of the basic principle of photosynthesis as a light‐induced redox process was unequivocally established by van Niel's work, along with that of other scientists of the time, notably Hans Gaffron (1902–1979).
3.7.2 The Hill reaction: separation of oxidation and reduction reactions
In the 1930s, Robert (Robin) Hill (1899–1991), working at Cambridge University, was able to separate and investigate individually the oxidation and reduction reactions of photosynthesis. This taking apart of a complex system into individual parts, and investigation of them in detail in the absence of the complexities of a living cell is a cornerstone of biochemistry, often called reductionism. Hill established that it was possible to restore high rates of oxygen evolution to chloroplast suspensions if the latter were supplied with any of a number of artificial electron acceptors (Hill, 1939). Initially, he used ferric salts, and the Fe3+ was reduced to Fe2+ through the action of light, at the same time producing O2. The reduction in artificial acceptors with concomitant O2 production is today known as the Hill reaction. An example of the Hill reaction is given in Eq. (3.6):