Nanotechnology in Plant Growth Promotion and Protection. Группа авторов
Чтение книги онлайн.
Читать онлайн книгу Nanotechnology in Plant Growth Promotion and Protection - Группа авторов страница 17
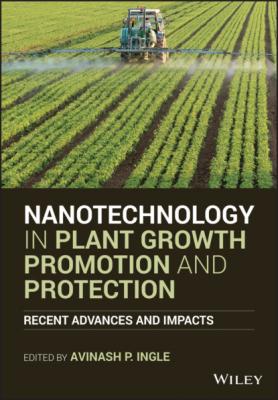
2.2 Properties of TiO2NPs Important for Biological Interaction
The methods used for the synthesis can profoundly change the properties of TiO2NPs that affect their biological interaction. Among the various properties, size and shape of TiO2NPs, their crystal structure, and surface coating are some of the most important properties found to affect the interaction of nanoparticles with other systems. Furthermore, the above‐mentioned properties also affect the surface area of nanoparticles, as well as their agglomeration/aggregation properties, generation of reactive oxygen species, and their ability to react with cell structures upon contact.
The size of the TiO2NPs has a twofold effect on the interaction with organisms. (1) Lower size corresponds to the higher surface area that can interact with the surfaces of organisms and thus negatively or positively affect the organism to a greater extent. Smaller nanoparticles were also observed to attach more easily to cell walls (Lin et al. 2014). The higher surface area can also generate more reactive oxygen species under sunlight illumination and thus more negatively affect the organism (Kim et al. 2014). An increase in malondialdehyde, another reactive compound was also observed to increase with decreasing size of TiO2NPs (Lin et al. 2014). (2) Particles of smaller size more readily pass through the epidermis and other membranes via pore structures. Thus, they can enter deeper and in larger concentrations into the organism and interact more strongly with plants' vital parts (Larue et al. 2012b). Also, it was reported that the pattern of accumulation in plant tissues may change depending on the size of nanoparticles. Larue et al. (2012a) observed that 14 nm TiO2NPs can easily accumulate in root parenchyma, whereas, 25 nm TiO2NPs accumulated primarily in the vascular cylinder. Overall, the smaller the size of the nanoparticles, the higher the toxicity observed at the same concentration (Wang and Fan 2014; Sun et al. 2015). There is a size limit to which nanoparticles have an effect on plants. Positive effects, such as germination and root elongation have been observed in smaller nanoparticles, but the same effect was not observed in larger particles and bulk TiO2. (Zheng et al. 2005; Larue et al. 2012a,b).
In plants, two thresholds for root exposure were suggested by Larue et al. (2012a) when experimenting on wheat: (1) TiO2NPs having a threshold diameter of 140 nm and above do not accumulate in roots, and (2) TiO2NPs having a threshold diameter of 36 nm can be accumulated in root parenchyma, but cannot translocate to plant parts above the ground. This threshold proposed for wheat can serve as an approximation for root uptake thresholds of other plants although there were some variations dependent on plant species (Larue et al. 2012b). When applied on leaves, there was a size‐exclusion limit higher than 100 nm in lettuce and they were internalized in parenchymatic tissues (Larue et al. 2014).
Not only the size of the TiO2 nanomaterials, but their shape is also very important. Nanospheres of TiO2 were found to be less toxic than other shapes, such as nanorods, nanowires, nanotubes, and nanobelts (Porter et al. 2012; Silva et al. 2013; Yeo and Nam 2013; Wang and Fan 2014; Landa et al. 2016). One of the proposed reasons is a higher surface area of the elongated shapes nanomaterials like nanorods and other similar shapes. Nevertheless, a study by Hsiao and Huang (2011) demonstrated that nanorods with the same surface area as that of TiO2 nanospheres showed higher toxicity, proposing the possible reason is that the area in contact with cells of an organism is more important than the whole surface area. However, some studies performed on plants show little to no difference between bulk TiO2, TiO2 nanospheres, and nanowires (Landa et al. 2016).
The surface of the TiO2NPs is strongly affected by their crystal structure and different crystal phases of TiO2 display varying properties. Four different crystal phases of TiO2 were synthesized in form of nanoparticles, that is, amorphous, anatase, rutile, and brookite. There was a consensus that the anatase phase produces more reactive oxygen species than both rutile and brookite. Brookite was the most inert out of the three (Lin et al. 2014; Wang and Fan 2014). However, amorphous TiO2 material was found to be even more toxic than anatase toward human lung epithelial cells (Hsiao and Huang 2011).
Because of the relative inertness of brookite, the toxicity of anatase and rutile is more studied. The precise mode of action of anatase and rutile may differ. Anatase nanoparticles damaged the nucleus and cell membrane of algal cells whereas rutile nanoparticles were reported to cause damages to chloroplast and internal organelles (Iswarya et al. 2015). Considering these facts, two different studies have been performed to study the effect of such crystal phases on cucumber plants. The results obtained revealed a preferential translocation of rutile nanoparticles over their anatase form in fruits, leaves, and phloem (Servin et al. 2012, 2013). Although the mode of action is different for both anatase and rutile phase nanoparticles, their toxicity toward microscopic algae differs only at higher concentrations, and anatase was found to be more toxic (Iswarya et al. 2015). Yet, no difference in toxicity was found in the case of higher plants (Larue et al. 2012b). There are also studies that challenge the notion that anatase is more toxic to plants, such as in the case of Silva et al. (2016, 2017) where anatase was found to be less toxic than a mixture of anatase and rutile.
Similarly, surface coating of nanoparticles can both increase or decrease toxicity toward organisms since the surface is the active region for nanoparticle interaction with biota and is determined by its chemical activity (Foltête et al. 2011; Wang and Fan 2014; Cox et al. 2016; Šebesta et al. 2019). The reports on comparing the influence of surface coating of TiO2NPs toward plants are relatively scarce. Tan et al. (2017) synthsezied TiO2NPs with a different surface coating such as unmodified, hydrophilic, and hydrophobic and evaluated their toxicity in basil (Ocimum basilicum). The findings recorded showed the differential toxicity for each type of nanoparticles in tested plants. Unlike hydrophobic TiO2NPs, unmodified and hydrophilic TiO2NPs had no negative effect on starch content and root elongation. The hydrophilic and hydrophobic TiO2NPs significantly reduced the seed germination and only unmodified and hydrophobic were reported to significantly decrease the shoot biomass. There were also differences in uptake of important nutrients and coated TiO2NPs had a greater effect on the nutritional quality of the plant (Tan et al. 2017). The surfactant in paints containing TiO2NPs was proposed to ease their internalization in plants (Larue et al. 2014).
2.3 Pathways and Interaction of TiO2NPs with Plants
Plants can be exposed to TiO2NPs both intentionally and unintentionally. Usually, there are three major pathways through which different plants can be exposed to TiO2NPs which include (1) foliar exposure, (2) root exposure, and (3) exposure to TiO2NPs after application on seeds (Figure 2.1). These pathways significantly affect the interaction of TiO2NPs with plants and their possible toxic or beneficial effects that these particles have on plants.
2.3.1 Foliar Exposure
Upon foliar application, TiO2NPs were taken up by leaves and were found in epidermis, parenchyma, and vascular tissues in both apoplastic and symplastic compartments (Larue et al. 2014). Several mechanisms have been proposed for underlying nanoparticle internalization. Both stomatal and cuticular pathways were suggested. TiO2NPs were found at stomatal openings, in sub‐stomatal chambers and vacuoles of guard cells (Kurepa et al. 2010; Larue et al. 2014). In addition, TiO2NPs were also reported to be entrapped in the cuticle thereby damaging it and the cell walls (Larue et al. 2014). Absorption through cell membranes probably occurred through endocytosis and TiO2NPs were observed in endosomes (Kurepa et al. 2010; Larue et al. 2012a).