Molecular Mechanisms of Photosynthesis. Robert E. Blankenship
Чтение книги онлайн.
Читать онлайн книгу Molecular Mechanisms of Photosynthesis - Robert E. Blankenship страница 32
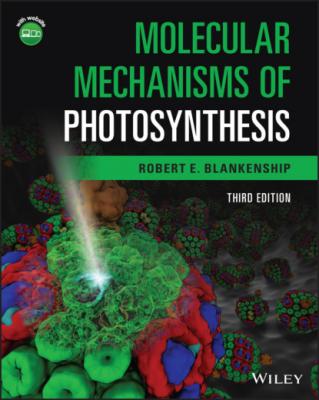
Figure 4.8 Absorption (left) and fluorescence (right) spectra of (a) chlorophyll a and (b) bacteriochlorophyll a in diethyl ether.
Figure 4.9 Molecular orbital energy level diagram of porphyrin, chlorin, and bacteriochlorin with one‐electron transitions that combine via configuration interaction to give the different electronic transitions indicated by arrows.
The diagram shown in Fig. 4.9 is an oversimplification of what is a complex relationship between electronic states and orbital energies. It is not correct to conclude that electronic transitions reflect a simple promotion of an electron from a HOMO to a LUMO. In reality, several different electronic configurations, including contributions from much higher‐energy molecular orbitals can contribute to the electronic transition. This phenomenon is known as configuration interaction. The result is that there is not a simple one‐to‐one correspondence between orbital occupations and electronic transitions. However, it is also the case that the majority contribution to the transition can come from a single configuration. This becomes more correct as one goes from the more symmetric porphyrin to the very asymmetric bacteriochlorin, so that the Qy absorption band in bacteriochlorophyll is described reasonably well by the simple HOMO to LUMO transition, while this is not valid for the more symmetrical porphyrin. The energies and electron densities associated with the four frontier molecular orbitals for a Zn‐chlorin are shown in Fig. 4.10. The one‐electron transitions that combine via configuration interaction to produce the excited electronic state are shown as arrows, with the major transitions that combine to produce the Qy transition labeled Y and those that combine to produce the Qx transition labeled X.
Figure 4.10 Schematic orbital energy diagram for a Znchlorin, illustrated by the calculated orbital energies (in eV) and electron density distributions. The diagram also shows the one‐electron promotions that give rise to the electronic states via configuration interaction according to the four‐orbital model.
Source: Kee et al. (2007) (p. 1137)/John Wiley and Sons.
The electronic transitions have transition dipole moments with different strengths and orientations. The longest‐wavelength transition is invariably polarized along the y‐axis of the molecule and is therefore known as the Qy transition. The y‐axis extends from the N atom in ring A to the N atom in ring C (Fig. 4.2). This means that the absorption will be strongest if the electric vector of plane‐polarized exciting light is parallel to the molecular axis of the pigment. The exciting light couples to the π electrons of the molecule and rearranges them somewhat during the transition. The Qy transition causes a shift in electron density that is directed along the y molecular axis of the molecule. In a similar fashion, the x‐axis extends from the N atom in ring B to the N atom in ring D.
For both chlorophyll a and bacteriochlorophyll a, the Qy transition is strongly polarized along the y molecular axis (see Appendix). The weaker Qx transition in bacteriochlorophyll is also strongly polarized along the x molecular axis. The Qx transition in chlorophyll, however, is not as well resolved as in bacteriochlorophyll, and theoretical calculations suggest that it is not polarized directly along the x molecular axis. The Soret bands have a mixed polarization.
In addition to the fundamental Q and B electronic transitions, vibrational overtone transitions can also be observed, especially on the Qy band. These represent a simultaneous vibrational and electronic transition, with the final state being an excited vibrational state of the excited electronic state. A progression of vibrational states can be observed, with the most intense transition the 0,0 band, with the higher energy satellites termed 0,1; 0,2; and so on. The first number is the vibrational quantum number of the ground electronic state before light absorption, and the second number the vibrational state of the excited electronic state after the transition. Of course, there are many vibrations in a chlorophyll molecule, and only one of these is the one responsible for the vibrational structure in the absorption spectrum.
The fluorescence spectrum of all chlorophylls peaks at slightly longer wavelengths than the absorption maximum. The fluorescence emission is polarized along the y molecular axis, as it is emitted from the Qy transition. The fluorescence spectrum usually has a characteristic “mirror image” relationship to the absorption. This is because the ground and excited states have similar shapes, so those molecular vibrations that are activated during electronic absorption are also likely to be activated upon fluorescence emission. However, in this case, the initial state is the ground vibrational state of the excited electronic state, and the final state is the excited vibrational state of the ground electronic state. This causes a shift of the emission to the longer‐wavelength side of the main transition, in what is known as the Stokes shift (see Appendix).
Table 4.2 brings together wavelengths of absorption maxima as well as molar extinction coefficients and fluorescence lifetimes and quantum yields of chlorophylls and bacteriochlorophylls in organic solvents. A comprehensive database of spectra and photophysical properties of chlorophylls and related pigments is available (Taniguchi and Lindsey, 2021). The spectral properties of these pigments are significantly altered when they are incorporated into protein complexes. In every case, the longest‐wavelength maximum shifts to longer wavelengths in pigment–proteins; sometimes the shift is more than 100 nm. We will examine the properties of these pigment–protein complexes in more detail in Chapters 5–7.
Table 4.2 Spectroscopic properties of chlorophylls and bacteriochlorophylls in vitroa
Source: Data from Scheer (1991) and Niedzwiedzki and Blankenship (2010).
Pigment | λ max (nm) | ɛ max (mM−1 cm−1) b | τ f (ns) | φ f |
---|---|---|---|---|
Chlorophyll a | 662, 578,430 | 90.0 | 6.3 |