Molecular Mechanisms of Photosynthesis. Robert E. Blankenship
Чтение книги онлайн.
Читать онлайн книгу Molecular Mechanisms of Photosynthesis - Robert E. Blankenship страница 30
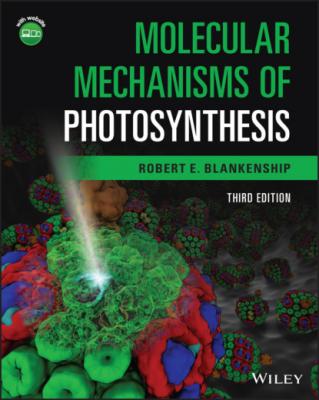
4.1.8 Bacteriochlorophyll b
Bacteriochlorophyll b is found only in a few species of purple bacteria. It differs from bacteriochlorophyll a only by the presence of an exocyclic double bond at C‐8 in ring B, which is called an ethylidine substituent. Its chemical structure is shown in Fig. 4.4. Bacteriochlorophyll b has the longest‐wavelength absorbance band of any known chlorophyll‐type pigment. in vivo, its absorbance maximum is at 960–1050 nm.
4.1.9 Bacteriochlorophylls c, d, e, and f
Bacteriochlorophylls c, d, e, and f will be considered as a group, because they are found only in green photosynthetic bacteria, organisms that contain the antenna complex known as a chlorosome. They are also unusual among chlorophylls in that they are invariably found as complex mixtures of closely related compounds instead of as a single compound of unique structure. Several distinct structural features are found in these pigments, whose structures are shown in Fig. 4.4. Ring B contains a C‐7–C‐8 double bond, as in chlorophylls, making these pigments chlorins instead of bacteriochlorins. They also have a hydroxyethyl substituent at the C‐31 position in ring A. This functional group is essential to the aggregation of these pigments in the chlorosome, which will be discussed in Chapter 5. The C‐31 carbon is chiral, and both R and S diastereomers are found in cells (the C‐17 and C‐18 chiral carbons are stereochemically pure). These pigments also have hydrogens at the C‐132 position, instead of the bulky carboxymethyl substituent found in all other chlorophylls. This change allows the chlorin rings to pack together more closely. These pigments are structurally programmed for aggregation, and indeed, in the chlorosome, they are found as large oligomeric complexes with little protein.
The differences among the bacteriochlorophylls c, d, e, and f occur primarily in the C‐20 methine bridge position, where bacteriochlorophylls c and e have a methyl substituent, and at the C‐7 position, where bacteriochlorophylls e and f have a formyl substituent, like chlorophyll b. These changes tune the light absorption properties of these pigments, with the wavelength of maximum absorption decreasing as one goes from bacteriochlorophyll c to f. Other differences are found at the C‐8 and C‐12 positions, where a complex variety of substituents can occur, even in a single organism. The tails of these bacteriochlorophylls are also different from those of most other chlorophylls. The bacteriochlorophylls c, d, and e found in the green sulfur bacteria contain a farnesol tail instead of a phytol. This is one isoprene unit shorter than phytol. The filamentous anoxygenic phototrophic bacteria, which contain only bacteriochlorophyll c (as well as bacteriochlorophyll a) primarily utilize the 18‐carbon straight‐chain stearol substituent, although a variety of other tails are found in varying amounts.
Bacteriochlorophyll f has never been found in nature. The compound that is known as bacteriochlorophyll f has the C‐7 formyl substituent of bacteriochlorophyll e, as well as the C‐20 H of bacteriochlorophyll d. It is thus the logical completion of this set of pigments. However, organisms that contain bacteriochlorophyll f have been created by inactivating the methylase enzyme that adds the methyl group to C‐20 (Vogl et al., 2012).
4.1.10 Bacteriochlorophyll g
Bacteriochlorophyll g is found only in the anoxygenic heliobacteria. Its structure is shown in Fig. 4.4. It is essentially a molecular hybrid of chlorophyll a and bacteriochlorophyll b, in that it contains the C‐3 vinyl substituent of chlorophyll a and the C‐8 exocyclic ethylidine substituent of bacteriochlorophyll b. It also contains a farnesyl tail instead of phytyl. Bacteriochlorophyll g is very unstable and isomerizes into chlorophyll a or closely related compounds.
4.2 Pheophytins and bacteriopheophytins
The metal‐free chlorophylls are known as pheophytins. The structures of pheophytin a and bacteriopheophytin a are shown in Fig. 4.5. In these compounds, two hydrogen ions replace the central Mg2+. Acidic conditions promote the displacement of the metal. Pheophytins are formed during the degradation of chlorophylls, so are often viewed as primarily breakdown products resulting from loss of the central metal. However, small amounts of pheophytin are an essential component of some reaction center complexes and are almost certainly made in a specific pathway, although it is not known. Pheophytin is somewhat easier to reduce than the corresponding chlorophyll, so it can function as an electron acceptor at a place in the sequence of electron carriers where chlorophyll will not work.
Figure 4.5 Chemical structures of pheophytin a and bacteriopheophytin a.
4.3 Chlorophyll biosynthesis
The chlorophyll biosynthetic pathway contains 17 enzymatic steps, as shown in Fig. 4.6. It begins with the formation of δ‐aminolevulinic acid (ALA) (Suzuki et al., 1997; Beale, 1999; Chew and Bryant, 2007; Masuda, 2008; Chen, 2014; Bryant et al., 2020). Eight molecules of ALA are condensed, eventually forming the symmetric metal‐free porphyrin: protoporphyrin IX. At this point the pathway branches, with one branch leading to heme and the other to chlorophyll, depending on whether Fe or Mg is incorporated. The chlorophyll branch includes additional steps in which the fifth ring is fashioned and a double bond in ring D is reduced to form chlorophyllide, which still lacks the isoprenoid tail. The isoprenoid tail is attached as the last step. Biosynthesis of bacteriochlorophyll a is identical to chlorophyll synthesis through the chlorophyllide a intermediate, but contains two additional steps: the reduction of ring B and the conversion of the vinyl group at C‐3 to an acetyl moiety. The order of the biosynthetic steps and the identities of most of the enzymes that carry out the transformations have been well established for both chlorophyll a and bacteriochlorophyll a synthesis by a combination of biochemical and genetic experiments. The biosynthetic pathway of chlorophylls is shown in Fig. 4.6. The enzymes that catalyze the various steps are identified in the figure legend.
The first few steps in the pathway produce ALA. Most photosynthetic organisms use a unique pathway that involves the ATP‐dependent charging of the amino acid glutamic acid to a glutamyl tRNA, the same reaction that takes place when glutamic acid is incorporated into a growing peptide during protein synthesis. This reaction is one of a very small number of reactions known in biology in which a tRNA molecule is used in a biochemical step other than protein synthesis. Step 2 is the NADPH‐dependent reductive cleavage of the acid to an aldehyde to form L‐glutamic acid 1‐semialdehyde. The final step in ALA synthesis is the transamination rearrangement to form ALA.
Surprisingly, one group of photosynthetic organisms makes ALA by a completely different route. This is the one‐step condensation of the amino acid glycine with succinyl‐CoA, followed