Autonomous Airborne Wireless Networks. Группа авторов
Чтение книги онлайн.
Читать онлайн книгу Autonomous Airborne Wireless Networks - Группа авторов страница 16
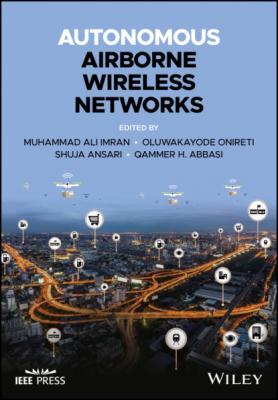
where
(2.15)
(2.16)
The path loss for LoS and NLoS links can be computed as
(2.18)
For a high‐altitude AG channel with
2.4.1.2 Small‐Scale Fading
Small‐scale fading refers to the random fluctuations of amplitude and phase of the received signal over a short distance or a short period of time due to constructive or destructive interference of the MPC. For different propagation environments and wireless systems, different distribution models are suggested to analyze the random variations in the received signal envelop. The Rician and Rayleigh distributions are widely used models in the literature of wireless communications, where both are based on the central limit theorem. The Rician distribution provides better fit for the AA and AG channels, where the impact of LoS propagation is stronger. On the other hand, when the MPC impinges at the receiver with random amplitude and phase, the small‐scale fading effect can be captured by the Rayleigh distribution [6].
Geometrical analysis, numerical simulations, and empirical data are used to obtain the stochastic fading models [38–40]. Geometry‐based stochastic channel model (GBSCM) is the most popular type of small‐scale fading model. GBSCM is subdivided into regular‐shaped geometry‐based stochastic channel model (RS‐GBSCM) and irregular‐shaped geometry‐based stochastic channel model (IS‐GBSCM). Time‐variant IS‐GBSCM was presented in [41] and RS‐GBSCM was presented in [42] and [43].These works illustrated Rician distribution for small‐scale fading. In [44], non‐geometric stochastic channel model (NGSCM) was provided, where small‐scale effects of AG propagation were modeled by using Rician and Loo models. Table 2.3 provides the measured characteristics of small‐scale fading of AG propagation in different environments.
Table 2.3 Measured small‐scale fading of AG propagation in different environments.
References | Scenario | Frequency band | Fading distribution |
---|---|---|---|
Khawaja et al. [11] | Suburban/Open field | Ultra‐wideband | Nakagami |
Newhall et al. [12] | Urban/Suburban | Wideband | Rayleigh, Rician |
Tu and Shimamoto [13] | Urban/Suburban | Wideband | Rician |
Matolak and Sun [14] | Urban/Suburban | Wideband | Rician |
Simunek et al. [45] | Urban/Suburban | Narrowband | Rician |
Cid et al. [46] | Forest/Foliage | Ultra‐wideband | Rician, Nakagami |
Matolak and Sun [47] | Sea/Fresh water | Wideband | Rician |
2.4.1.3 Airframe Shadowing
Airframe shadowing occurs when the LoS of AG propagation is obstructed by the UAV structure. This impairment is unique to UAV communications for both AA and AG channels and does not exist in conventional cellular communications. Airframe shadowing is more severe in fixed‐wing UAVs mounted with single antenna. In this case, the AG communication link can be severe during roll, pitch, or yaw motion of the UAV. One possible solution to alleviate airframe shadowing is to replace the single‐antenna system with spatially separated multiple antennas. Other factors responsible for airframe shadowing are the size, shape, and material of the UAV. The seminal work on the measurement of airframe shadowing was performed in [48], which found that the aircraft roll angle was proportional to the shadowing attenuation. Moreover, shadowing duration depends on the flight maneuvering.
2.5 Key Research Challenges of UAV‐Enabled Wireless Network
This section discusses some of the key research challenges for the practical deployment of UAVs as airborne wireless nodes.
2.5.1 Optimal Deployment of UAVs
In UAV‐based communications, one of the key challenges is the optimal three‐dimensional deployment of hovering UAV. The capability of UAV to maneuver and adjust its altitude provides additional degree of freedom for UAV deployment in an efficient manner to improve capacity and coverage. In fact, UAV deployment is more challenging in UAV communications than in conventional terrestrial communications because the characteristics of AG propagation change with the position of the UAV. However, for efficient UAV deployment, flight duration and energy constraints must be taken into account for battery‐operated UAV, as they affect the performance of networks. In addition, simultaneous deployment of multiple UAVs is more challenging because of the co‐channel interference and the possibility of airborne collision of UAVs. Another important issue is the UAV deployment in the presence of terrestrial network. UAV deployment problem has been extensively discussed in the literature for coverage maximization 17,29,30,33,33, data collection from Internet of Things (IoT) devices [31], UAV‐assisted wireless network [27], disaster scenario [49], and caching applications [22].
2.5.2 UAV Trajectory Optimization
Optimal